The 19S proteasome activator promotes human cytomegalovirus immediate early gene expression through proteolytic and nonproteolytic mechanisms
- PMID: 25078702
- PMCID: PMC4178716
- DOI: 10.1128/JVI.01720-14
The 19S proteasome activator promotes human cytomegalovirus immediate early gene expression through proteolytic and nonproteolytic mechanisms
Abstract
Proteasomes are large, multisubunit complexes that support normal cellular activities by executing the bulk of protein turnover. During infection, many viruses have been shown to promote viral replication by using proteasomes to degrade cellular factors that restrict viral replication. For example, the human cytomegalovirus (HCMV) pp71 protein induces the proteasomal degradation of Daxx, a cellular transcriptional repressor that can silence viral immediate early (IE) gene expression. We previously showed that this degradation requires both the proteasome catalytic 20S core particle (CP) and the 19S regulatory particle (RP). The 19S RP associates with the 20S CP to facilitate protein degradation but also plays a 20S CP-independent role promoting transcription. Here, we present a nonproteolytic role of the 19S RP in HCMV IE gene expression. We demonstrate that 19S RP subunits are recruited to the major immediate early promoter (MIEP) that directs IE transcription. Depletion of 19S RP subunits generated a defect in RNA polymerase II elongation through the MIE locus during HCMV infection. Our results reveal that HCMV commandeers proteasome components for both proteolytic and nonproteolytic roles to promote HCMV lytic infection. Importance: Proteasome inhibitors decrease or eliminate 20S CP activity and are garnering increasing interest as chemotherapeutics. However, an increasing body of evidence implicates 19S RP subunits in important proteolytic-independent roles during transcription. Thus, pharmacological inhibition of the 20S CP as a means to modulate proteasome function toward therapeutic effect is an incomplete capitalization on the potential of this approach. Here, we provide an additional example of nonproteolytic 19S RP function in promoting HCMV transcription. These data provide a novel system with which to study the roles of different proteasome components during transcription, a rationale for previously described shifts in 19S RP subunit localization during HCMV infection, and a potential therapeutic intervention point at a pre-immediate early stage for the inhibition of HCMV infection.
Copyright © 2014, American Society for Microbiology. All Rights Reserved.
Figures
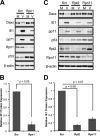
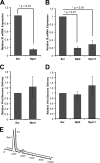
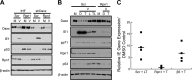
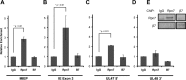
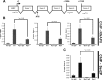
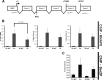
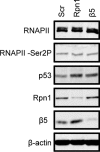
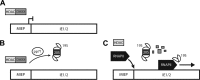
Similar articles
-
Ubiquitin-independent proteasomal degradation of tumor suppressors by human cytomegalovirus pp71 requires the 19S regulatory particle.J Virol. 2013 Apr;87(8):4665-71. doi: 10.1128/JVI.03301-12. Epub 2013 Feb 13. J Virol. 2013. PMID: 23408605 Free PMC article.
-
Proteasome subunits relocalize during human cytomegalovirus infection, and proteasome activity is necessary for efficient viral gene transcription.J Virol. 2010 Mar;84(6):3079-93. doi: 10.1128/JVI.02236-09. Epub 2009 Dec 30. J Virol. 2010. PMID: 20042513 Free PMC article.
-
Method of Monitoring 26S Proteasome in Cells Revealed the Crucial Role of PSMA3 C-Terminus in 26S Integrity.Biomolecules. 2023 Jun 15;13(6):992. doi: 10.3390/biom13060992. Biomolecules. 2023. PMID: 37371572 Free PMC article.
-
Small-Molecule Inhibitors of the Proteasome's Regulatory Particle.Chembiochem. 2019 Jul 15;20(14):1739-1753. doi: 10.1002/cbic.201900017. Epub 2019 May 24. Chembiochem. 2019. PMID: 30740849 Free PMC article. Review.
-
Order of the proteasomal ATPases and eukaryotic proteasome assembly.Cell Biochem Biophys. 2011 Jun;60(1-2):13-20. doi: 10.1007/s12013-011-9178-4. Cell Biochem Biophys. 2011. PMID: 21461838 Free PMC article. Review.
Cited by
-
Canonical and Variant Forms of Histone H3 Are Deposited onto the Human Cytomegalovirus Genome during Lytic and Latent Infections.J Virol. 2016 Oct 28;90(22):10309-10320. doi: 10.1128/JVI.01220-16. Print 2016 Nov 15. J Virol. 2016. PMID: 27605676 Free PMC article.
-
A virally encoded high-resolution screen of cytomegalovirus dependencies.Nature. 2024 Jun;630(8017):712-719. doi: 10.1038/s41586-024-07503-z. Epub 2024 Jun 5. Nature. 2024. PMID: 38839957
-
NFκB and Cyclic AMP Response Element Sites Mediate the Valproic Acid and UL138 Responsiveness of the Human Cytomegalovirus Major Immediate Early Enhancer and Promoter.J Virol. 2023 Mar 30;97(3):e0002923. doi: 10.1128/jvi.00029-23. Epub 2023 Mar 1. J Virol. 2023. PMID: 36856444 Free PMC article.
-
Non-proteolytic activity of 19S proteasome subunit RPT-6 regulates GATA transcription during response to infection.PLoS Genet. 2018 Sep 28;14(9):e1007693. doi: 10.1371/journal.pgen.1007693. eCollection 2018 Sep. PLoS Genet. 2018. PMID: 30265660 Free PMC article.
-
cGAS-STING-TBK1 Signaling Promotes Valproic Acid-Responsive Human Cytomegalovirus Immediate-Early Transcription during Infection of Incompletely Differentiated Myeloid Cells.Viruses. 2024 May 30;16(6):877. doi: 10.3390/v16060877. Viruses. 2024. PMID: 38932169 Free PMC article.
References
Publication types
MeSH terms
Substances
Grants and funding
LinkOut - more resources
Full Text Sources
Other Literature Sources
Miscellaneous