Acute hypoxia affects P-TEFb through HDAC3 and HEXIM1-dependent mechanism to promote gene-specific transcriptional repression
- PMID: 25056306
- PMCID: PMC4132729
- DOI: 10.1093/nar/gku611
Acute hypoxia affects P-TEFb through HDAC3 and HEXIM1-dependent mechanism to promote gene-specific transcriptional repression
Abstract
Hypoxia is associated with a variety of physiological and pathological conditions and elicits specific transcriptional responses. The elongation competence of RNA Polymerase II is regulated by the positive transcription elongation factor b (P-TEFb)-dependent phosphorylation of Ser2 residues on its C-terminal domain. Here, we report that hypoxia inhibits transcription at the level of elongation. The mechanism involves enhanced formation of inactive complex of P-TEFb with its inhibitor HEXIM1 in an HDAC3-dependent manner. Microarray transcriptome profiling of hypoxia primary response genes identified ∼79% of these genes being HEXIM1-dependent. Hypoxic repression of P-TEFb was associated with reduced acetylation of its Cdk9 and Cyclin T1 subunits. Hypoxia caused nuclear translocation and co-localization of the Cdk9 and HDAC3/N-CoR repressor complex. We demonstrated that the described mechanism is involved in hypoxic repression of the monocyte chemoattractant protein-1 (MCP-1) gene. Thus, HEXIM1 and HDAC-dependent deacetylation of Cdk9 and Cyclin T1 in response to hypoxia signalling alters the P-TEFb functional equilibrium, resulting in repression of transcription.
© The Author(s) 2014. Published by Oxford University Press on behalf of Nucleic Acids Research.
Figures
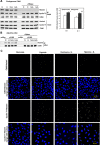
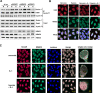
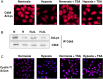
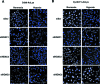
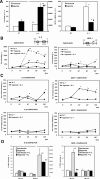
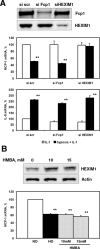
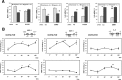
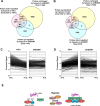
Similar articles
-
Regulation of P-TEFb elongation complex activity by CDK9 acetylation.Mol Cell Biol. 2007 Jul;27(13):4641-51. doi: 10.1128/MCB.00857-06. Epub 2007 Apr 23. Mol Cell Biol. 2007. PMID: 17452463 Free PMC article.
-
T-loop phosphorylated Cdk9 localizes to nuclear speckle domains which may serve as sites of active P-TEFb function and exchange between the Brd4 and 7SK/HEXIM1 regulatory complexes.J Cell Physiol. 2010 Jul;224(1):84-93. doi: 10.1002/jcp.22096. J Cell Physiol. 2010. PMID: 20201073 Free PMC article.
-
Release of positive transcription elongation factor b (P-TEFb) from 7SK small nuclear ribonucleoprotein (snRNP) activates hexamethylene bisacetamide-inducible protein (HEXIM1) transcription.J Biol Chem. 2014 Apr 4;289(14):9918-25. doi: 10.1074/jbc.M113.539015. Epub 2014 Feb 10. J Biol Chem. 2014. PMID: 24515107 Free PMC article.
-
P-TEFb: The master regulator of transcription elongation.Mol Cell. 2023 Feb 2;83(3):393-403. doi: 10.1016/j.molcel.2022.12.006. Epub 2023 Jan 3. Mol Cell. 2023. PMID: 36599353 Free PMC article. Review.
-
CYCLINg through transcription: posttranslational modifications of P-TEFb regulate transcription elongation.Cell Cycle. 2010 May;9(9):1697-705. doi: 10.4161/cc.9.9.11346. Epub 2010 May 29. Cell Cycle. 2010. PMID: 20436276 Free PMC article. Review.
Cited by
-
Epigenetics: A Potential Mechanism Involved in the Pathogenesis of Various Adverse Consequences of Obstructive Sleep Apnea.Int J Mol Sci. 2019 Jun 15;20(12):2937. doi: 10.3390/ijms20122937. Int J Mol Sci. 2019. PMID: 31208080 Free PMC article. Review.
-
Hypoxia and Chromatin: A Focus on Transcriptional Repression Mechanisms.Biomedicines. 2018 Apr 22;6(2):47. doi: 10.3390/biomedicines6020047. Biomedicines. 2018. PMID: 29690561 Free PMC article. Review.
-
Bacterial Short Chain Fatty Acids Push All The Buttons Needed To Reactivate Latent Viruses.Stem Cell Epigenet. 2015;2(1):e532. doi: 10.14800/sce.532. Stem Cell Epigenet. 2015. PMID: 26029743 Free PMC article.
-
Acetylation and Phosphorylation in the Regulation of Hypoxia-Inducible Factor Activities: Additional Options to Modulate Adaptations to Changes in Oxygen Levels.Life (Basel). 2023 Dec 21;14(1):20. doi: 10.3390/life14010020. Life (Basel). 2023. PMID: 38276269 Free PMC article. Review.
-
Lysine Acetylation Goes Global: From Epigenetics to Metabolism and Therapeutics.Chem Rev. 2018 Feb 14;118(3):1216-1252. doi: 10.1021/acs.chemrev.7b00181. Epub 2018 Feb 6. Chem Rev. 2018. PMID: 29405707 Free PMC article. Review.
References
-
- Mohyeldin A., Garzon-Muvdi T., Quinones-Hinojosa A. Oxygen in stem cell biology: a critical component of the stem cell niche. Cell Stem Cell. 2010;7:150–161. - PubMed
-
- Safronova O., Morita I. Transcriptome remodeling in hypoxic inflammation. J. Dent. Res. 2010;89:430–444. - PubMed
-
- Safronova O., Pluemsampant S., Nakahama K., Morita I. Regulation of chemokine gene expression by hypoxia via cooperative activation of NF-kappaB and histone deacetylase. Int. J. Biochem. Cell Biol. 2009;41:2270–2280. - PubMed
-
- Aoi Y., Nakahama K., Morita I., Safronova O. The involvement of DNA and histone methylation in the repression of IL-1beta-induced MCP-1 production by hypoxia. Biochem. Biophys. Res. Commun. 2011;414:252–258. - PubMed
-
- Safronova O., Nakahama K., Onodera M., Muneta T., Morita I. Effect of hypoxia on monocyte chemotactic protein-1 (MCP-1) gene expression induced by Interleukin-1beta in human synovial fibroblasts. Inflamm. Res. 2003;52:480–486. - PubMed
Publication types
MeSH terms
Substances
LinkOut - more resources
Full Text Sources
Other Literature Sources
Molecular Biology Databases
Research Materials
Miscellaneous