Transfer RNA and human disease
- PMID: 24917879
- PMCID: PMC4042891
- DOI: 10.3389/fgene.2014.00158
Transfer RNA and human disease
Abstract
Pathological mutations in tRNA genes and tRNA processing enzymes are numerous and result in very complicated clinical phenotypes. Mitochondrial tRNA (mt-tRNA) genes are "hotspots" for pathological mutations and over 200 mt-tRNA mutations have been linked to various disease states. Often these mutations prevent tRNA aminoacylation. Disrupting this primary function affects protein synthesis and the expression, folding, and function of oxidative phosphorylation enzymes. Mitochondrial tRNA mutations manifest in a wide panoply of diseases related to cellular energetics, including COX deficiency (cytochrome C oxidase), mitochondrial myopathy, MERRF (Myoclonic Epilepsy with Ragged Red Fibers), and MELAS (mitochondrial encephalomyopathy, lactic acidosis, and stroke-like episodes). Diseases caused by mt-tRNA mutations can also affect very specific tissue types, as in the case of neurosensory non-syndromic hearing loss and pigmentary retinopathy, diabetes mellitus, and hypertrophic cardiomyopathy. Importantly, mitochondrial heteroplasmy plays a role in disease severity and age of onset as well. Not surprisingly, mutations in enzymes that modify cytoplasmic and mitochondrial tRNAs are also linked to a diverse range of clinical phenotypes. In addition to compromised aminoacylation of the tRNAs, mutated modifying enzymes can also impact tRNA expression and abundance, tRNA modifications, tRNA folding, and even tRNA maturation (e.g., splicing). Some of these pathological mutations in tRNAs and processing enzymes are likely to affect non-canonical tRNA functions, and contribute to the diseases without significantly impacting on translation. This chapter will review recent literature on the relation of mitochondrial and cytoplasmic tRNA, and enzymes that process tRNAs, to human disease. We explore the mechanisms involved in the clinical presentation of these various diseases with an emphasis on neurological disease.
Keywords: Usher syndrome Type IIIB; aminoacyl-tRNA synthetase; localized translation; mitochondrial disease; neurodegenerative disease; tRNA.
Figures
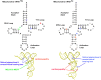
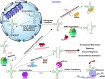
Similar articles
-
Human mitochondrial leucyl-tRNA synthetase corrects mitochondrial dysfunctions due to the tRNALeu(UUR) A3243G mutation, associated with mitochondrial encephalomyopathy, lactic acidosis, and stroke-like symptoms and diabetes.Mol Cell Biol. 2010 May;30(9):2147-54. doi: 10.1128/MCB.01614-09. Epub 2010 Mar 1. Mol Cell Biol. 2010. PMID: 20194621 Free PMC article.
-
Nuclear-encoded factors involved in post-transcriptional processing and modification of mitochondrial tRNAs in human disease.Front Genet. 2015 Mar 10;6:79. doi: 10.3389/fgene.2015.00079. eCollection 2015. Front Genet. 2015. PMID: 25806043 Free PMC article. Review.
-
Correction of the consequences of mitochondrial 3243A>G mutation in the MT-TL1 gene causing the MELAS syndrome by tRNA import into mitochondria.Nucleic Acids Res. 2011 Oct;39(18):8173-86. doi: 10.1093/nar/gkr546. Epub 2011 Jun 30. Nucleic Acids Res. 2011. PMID: 21724600 Free PMC article.
-
Human mitochondrial tRNAs: biogenesis, function, structural aspects, and diseases.Annu Rev Genet. 2011;45:299-329. doi: 10.1146/annurev-genet-110410-132531. Epub 2011 Sep 6. Annu Rev Genet. 2011. PMID: 21910628 Review.
-
Human mitochondrial diseases caused by lack of taurine modification in mitochondrial tRNAs.Wiley Interdiscip Rev RNA. 2011 May-Jun;2(3):376-86. doi: 10.1002/wrna.65. Epub 2011 Feb 25. Wiley Interdiscip Rev RNA. 2011. PMID: 21957023 Review.
Cited by
-
tRF2Cancer: A web server to detect tRNA-derived small RNA fragments (tRFs) and their expression in multiple cancers.Nucleic Acids Res. 2016 Jul 8;44(W1):W185-93. doi: 10.1093/nar/gkw414. Epub 2016 May 13. Nucleic Acids Res. 2016. PMID: 27179031 Free PMC article.
-
Codon optimality-mediated mRNA degradation: Linking translational elongation to mRNA stability.Mol Cell. 2022 Apr 21;82(8):1467-1476. doi: 10.1016/j.molcel.2022.03.032. Mol Cell. 2022. PMID: 35452615 Free PMC article. Review.
-
Small but large enough: structural properties of armless mitochondrial tRNAs from the nematode Romanomermis culicivorax.Nucleic Acids Res. 2018 Sep 28;46(17):9170-9180. doi: 10.1093/nar/gky593. Nucleic Acids Res. 2018. PMID: 29986062 Free PMC article.
-
Independent and sensory human mitochondrial functions reflecting symbiotic evolution.Front Cell Infect Microbiol. 2023 Jun 14;13:1130197. doi: 10.3389/fcimb.2023.1130197. eCollection 2023. Front Cell Infect Microbiol. 2023. PMID: 37389212 Free PMC article. Review.
-
PON-mt-tRNA: a multifactorial probability-based method for classification of mitochondrial tRNA variations.Nucleic Acids Res. 2016 Mar 18;44(5):2020-7. doi: 10.1093/nar/gkw046. Epub 2016 Feb 3. Nucleic Acids Res. 2016. PMID: 26843426 Free PMC article.
References
Publication types
Grants and funding
LinkOut - more resources
Full Text Sources
Other Literature Sources