Rac1 GTPase silencing counteracts microgravity-induced effects on osteoblastic cells
- PMID: 24903274
- PMCID: PMC5395736
- DOI: 10.1096/fj.14-249714
Rac1 GTPase silencing counteracts microgravity-induced effects on osteoblastic cells
Abstract
Bone cells exposed to real microgravity display alterations of their cytoskeleton and focal adhesions, two major mechanosensitive structures. These structures are controlled by small GTPases of the Ras homology (Rho) family. We investigated the effects of RhoA, Rac1, and Cdc42 modulation of osteoblastic cells under microgravity conditions. Human MG-63 osteoblast-like cells silenced for RhoGTPases were cultured in the automated Biobox bioreactor (European Space Agency) aboard the Foton M3 satellite and compared to replicate ground-based controls. The cells were fixed after 69 h of microgravity exposure for postflight analysis of focal contacts, F-actin polymerization, vascular endothelial growth factor (VEGF) expression, and matrix targeting. We found that RhoA silencing did not affect sensitivity to microgravity but that Rac1 and, to a lesser extent, Cdc42 abrogation was particularly efficient in counteracting the spaceflight-related reduction of the number of focal contacts [-50% in silenced, scrambled (SiScr) controls vs. -15% for SiRac1], the number of F-actin fibers (-60% in SiScr controls vs. -10% for SiRac1), and the depletion of matrix-bound VEGF (-40% in SiScr controls vs. -8% for SiRac1). Collectively, these data point out the role of the VEGF/Rho GTPase axis in mechanosensing and validate Rac1-mediated signaling pathways as potential targets for counteracting microgravity effects.
Keywords: Cdc42; MG-63; RhoA; VEGF; spaceflight.
© FASEB.
Figures
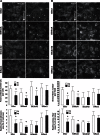
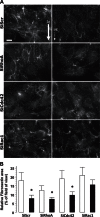
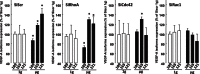
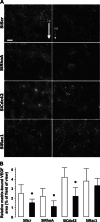
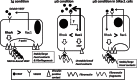
Similar articles
-
RhoA, Rac1, and Cdc42 exert distinct effects on epithelial barrier via selective structural and biochemical modulation of junctional proteins and F-actin.Am J Physiol Cell Physiol. 2004 Aug;287(2):C327-35. doi: 10.1152/ajpcell.00087.2004. Epub 2004 Mar 24. Am J Physiol Cell Physiol. 2004. PMID: 15044152
-
Vav2 activates Rac1, Cdc42, and RhoA downstream from growth factor receptors but not beta1 integrins.Mol Cell Biol. 2000 Oct;20(19):7160-9. doi: 10.1128/MCB.20.19.7160-7169.2000. Mol Cell Biol. 2000. PMID: 10982832 Free PMC article.
-
Rac and Rho play opposing roles in the regulation of hypoxia/reoxygenation-induced permeability changes in pulmonary artery endothelial cells.Am J Physiol Lung Cell Mol Physiol. 2005 Apr;288(4):L749-60. doi: 10.1152/ajplung.00361.2004. Epub 2004 Dec 10. Am J Physiol Lung Cell Mol Physiol. 2005. PMID: 15591411
-
Function of the cytoskeleton in gravisensing during spaceflight.Adv Space Res. 2003;32(8):1585-93. doi: 10.1016/S0273-1177(03)90399-1. Adv Space Res. 2003. PMID: 15002415 Review.
-
Driving Rho GTPase activity in endothelial cells regulates barrier integrity.Thromb Haemost. 2010 Jan;103(1):40-55. doi: 10.1160/TH09-06-0403. Epub 2009 Sep 30. Thromb Haemost. 2010. PMID: 20062930 Review.
Cited by
-
RhoGTPase stimulation is associated with strontium chloride treatment to counter simulated microgravity-induced changes in multipotent cell commitment.NPJ Microgravity. 2017 Jan 25;3:7. doi: 10.1038/s41526-016-0004-6. eCollection 2017. NPJ Microgravity. 2017. PMID: 28649629 Free PMC article.
-
RhoGTPases as key players in mammalian cell adaptation to microgravity.Biomed Res Int. 2015;2015:747693. doi: 10.1155/2015/747693. Epub 2015 Jan 29. Biomed Res Int. 2015. PMID: 25649831 Free PMC article. Review.
-
Microgravity triggers ferroptosis and accelerates senescence in the MG-63 cell model of osteoblastic cells.NPJ Microgravity. 2023 Dec 16;9(1):91. doi: 10.1038/s41526-023-00339-3. NPJ Microgravity. 2023. PMID: 38104197 Free PMC article.
-
Spaceflight-Induced Bone Tissue Changes that Affect Bone Quality and Increase Fracture Risk.Curr Osteoporos Rep. 2020 Feb;18(1):1-12. doi: 10.1007/s11914-019-00540-y. Curr Osteoporos Rep. 2020. PMID: 31897866 Review.
-
The role of wnt signaling in diabetes-induced osteoporosis.Diabetol Metab Syndr. 2023 Apr 28;15(1):84. doi: 10.1186/s13098-023-01067-0. Diabetol Metab Syndr. 2023. PMID: 37106471 Free PMC article. Review.
References
-
- Vico L., Collet P., Guignandon A., Lafage-Proust M. H., Thomas T., Rehaillia M., Alexandre C. (2000) Effects of long-term microgravity exposure on cancellous and cortical weight-bearing bones of cosmonauts. Lancet 355, 1607–1611 - PubMed
-
- Carmeliet G., Bouillon R. (1999) The effect of microgravity on morphology and gene expression of osteoblasts in vitro. FASEB J. 13(Suppl.), S129–S134 - PubMed
-
- Burger E. H., Klein-Nulend J. (1998) Microgravity and bone cell mechanosensitivity. Bone 22, 127S–130S - PubMed
-
- Hughes-Fulford M. (2003) Function of the cytoskeleton in gravisensing during spaceflight. Adv. Space Res. 32, 1585–1593 - PubMed
-
- Nabavi N., Khandani A., Camirand A., Harrison R. E. (2011) Effects of microgravity on osteoclast bone resorption and osteoblast cytoskeletal organization and adhesion. Bone 49, 965–974 - PubMed
Publication types
MeSH terms
Substances
LinkOut - more resources
Full Text Sources
Other Literature Sources
Research Materials
Miscellaneous