Budding yeast for budding geneticists: a primer on the Saccharomyces cerevisiae model system
- PMID: 24807111
- PMCID: PMC4012490
- DOI: 10.1534/genetics.114.163188
Budding yeast for budding geneticists: a primer on the Saccharomyces cerevisiae model system
Abstract
The budding yeast Saccharomyces cerevisiae is a powerful model organism for studying fundamental aspects of eukaryotic cell biology. This Primer article presents a brief historical perspective on the emergence of this organism as a premier experimental system over the course of the past century. An overview of the central features of the S. cerevisiae genome, including the nature of its genetic elements and general organization, is also provided. Some of the most common experimental tools and resources available to yeast geneticists are presented in a way designed to engage and challenge undergraduate and graduate students eager to learn more about the experimental amenability of budding yeast. Finally, a discussion of several major discoveries derived from yeast studies highlights the far-reaching impact that the yeast system has had and will continue to have on our understanding of a variety of cellular processes relevant to all eukaryotes, including humans.
Keywords: Saccharomyces cerevisiae; education; primer; yeast.
Figures
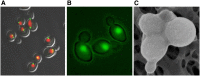
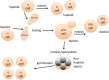
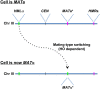
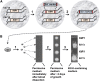
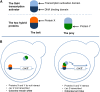
Similar articles
-
An Ancient Yeast for Young Geneticists: A Primer on the Schizosaccharomyces pombe Model System.Genetics. 2015 Oct;201(2):403-23. doi: 10.1534/genetics.115.181503. Genetics. 2015. PMID: 26447128 Free PMC article. Review.
-
Dynamic Live Cell Imaging of Budding Yeast Meiosis.Methods Mol Biol. 2024;2818:161-169. doi: 10.1007/978-1-0716-3906-1_10. Methods Mol Biol. 2024. PMID: 39126473
-
Screening the budding yeast genome reveals unique factors affecting K2 toxin susceptibility.PLoS One. 2012;7(12):e50779. doi: 10.1371/journal.pone.0050779. Epub 2012 Dec 5. PLoS One. 2012. PMID: 23227207 Free PMC article.
-
Naumovozyma castellii: an alternative model for budding yeast molecular biology.Yeast. 2017 Mar;34(3):95-109. doi: 10.1002/yea.3218. Epub 2016 Dec 2. Yeast. 2017. PMID: 27794167 Review.
-
The reference genome sequence of Saccharomyces cerevisiae: then and now.G3 (Bethesda). 2014 Mar 20;4(3):389-98. doi: 10.1534/g3.113.008995. G3 (Bethesda). 2014. PMID: 24374639 Free PMC article.
Cited by
-
Exploring the Molecular Underpinnings of Cancer-Causing Oncohistone Mutants Using Yeast as a Model.J Fungi (Basel). 2023 Dec 11;9(12):1187. doi: 10.3390/jof9121187. J Fungi (Basel). 2023. PMID: 38132788 Free PMC article. Review.
-
Saccharomyces cerevisiae Requires CFF1 To Produce 4-Hydroxy-5-Methylfuran-3(2H)-One, a Mimic of the Bacterial Quorum-Sensing Autoinducer AI-2.mBio. 2021 Mar 9;12(2):e03303-20. doi: 10.1128/mBio.03303-20. mBio. 2021. PMID: 33688008 Free PMC article.
-
Chemogenetic interactions in human cancer cells.Comput Struct Biotechnol J. 2019 Oct 26;17:1318-1325. doi: 10.1016/j.csbj.2019.09.006. eCollection 2019. Comput Struct Biotechnol J. 2019. PMID: 31921397 Free PMC article. Review.
-
Use of Haploid Model of Candida albicans to Uncover Mechanism of Action of a Novel Antifungal Agent.Front Cell Infect Microbiol. 2018 Jun 8;8:164. doi: 10.3389/fcimb.2018.00164. eCollection 2018. Front Cell Infect Microbiol. 2018. PMID: 29938200 Free PMC article.
-
Membrane protein-based biosensors.J R Soc Interface. 2018 Apr;15(141):20170952. doi: 10.1098/rsif.2017.0952. J R Soc Interface. 2018. PMID: 29669891 Free PMC article. Review.
References
-
- Asturias F. J., Jiang Y. W., Myers L. C., Gustafsson C. M., Kornberg R. D., 1999. Conserved structures of mediator and RNA polymerase II holoenzyme. Science 283: 985–987. - PubMed
-
- Baker D., Hicke L., Rexach M., Schleyer M., Schekman R., 1988. Reconstitution of SEC gene product-dependent intercompartmental protein transport. Cell 54: 335–344. - PubMed
-
- Barlowe C., Orci L., Yeung T., Hosobuchi M., Hamamoto S., et al. , 1994. COPII: a membrane coat formed by Sec proteins that drive vesicle budding from the endoplasmic reticulum. Cell 77: 895–907. - PubMed
-
- Blackburn E. H., 1984. The molecular structure of centromeres and telomeres. Annu. Rev. Biochem. 53: 163–194. - PubMed
-
- Blackburn E. H., Gall J. G., 1978. A tandemly repeated sequence at the termini of the extrachromosomal ribosomal RNA genes in Tetrahymena. J. Mol. Biol. 120: 33–53. - PubMed
Publication types
MeSH terms
LinkOut - more resources
Full Text Sources
Other Literature Sources
Molecular Biology Databases
Miscellaneous