Auxiliary tRNAs: large-scale analysis of tRNA genes reveals patterns of tRNA repertoire dynamics
- PMID: 24782525
- PMCID: PMC4041420
- DOI: 10.1093/nar/gku245
Auxiliary tRNAs: large-scale analysis of tRNA genes reveals patterns of tRNA repertoire dynamics
Abstract
Decoding of all codons can be achieved by a subset of tRNAs. In bacteria, certain tRNA species are mandatory, while others are auxiliary and are variably used. It is currently unknown how this variability has evolved and whether it provides an adaptive advantage. Here we shed light on the subset of auxiliary tRNAs, using genomic data from 319 bacteria. By reconstructing the evolution of tRNAs we show that the auxiliary tRNAs are highly dynamic, being frequently gained and lost along the phylogenetic tree, with a clear dominance of loss events for most auxiliary tRNA species. We reveal distinct co-gain and co-loss patterns for subsets of the auxiliary tRNAs, suggesting that they are subjected to the same selection forces. Controlling for phylogenetic dependencies, we find that the usage of these tRNA species is positively correlated with GC content and may derive directly from nucleotide bias or from preference of Watson-Crick codon-anticodon interactions. Our results highlight the highly dynamic nature of these tRNAs and their complicated balance with codon usage.
© The Author(s) 2014. Published by Oxford University Press on behalf of Nucleic Acids Research.
Figures
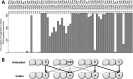
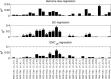
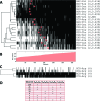
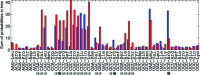
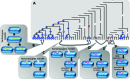
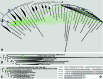
Similar articles
-
The influence of anticodon-codon interactions and modified bases on codon usage bias in bacteria.Mol Biol Evol. 2010 Sep;27(9):2129-40. doi: 10.1093/molbev/msq102. Epub 2010 Apr 19. Mol Biol Evol. 2010. PMID: 20403966
-
Codon usage bias from tRNA's point of view: redundancy, specialization, and efficient decoding for translation optimization.Genome Res. 2004 Nov;14(11):2279-86. doi: 10.1101/gr.2896904. Epub 2004 Oct 12. Genome Res. 2004. PMID: 15479947 Free PMC article.
-
Wobbling Forth and Drifting Back: The Evolutionary History and Impact of Bacterial tRNA Modifications.Mol Biol Evol. 2018 Aug 1;35(8):2046-2059. doi: 10.1093/molbev/msy110. Mol Biol Evol. 2018. PMID: 29846694 Free PMC article.
-
Repertoires of tRNAs: The Couplers of Genomics and Proteomics.Annu Rev Cell Dev Biol. 2018 Oct 6;34:239-264. doi: 10.1146/annurev-cellbio-100617-062754. Epub 2018 Aug 20. Annu Rev Cell Dev Biol. 2018. PMID: 30125138 Review.
-
Preferential codon usage in prokaryotic genes: the optimal codon-anticodon interaction energy and the selective codon usage in efficiently expressed genes.Gene. 1982 Jun;18(3):199-209. doi: 10.1016/0378-1119(82)90157-3. Gene. 1982. PMID: 6751939 Review.
Cited by
-
Determining the effects of temperature on the evolution of bacterial tRNA pools.bioRxiv [Preprint]. 2023 Oct 9:2023.09.26.559538. doi: 10.1101/2023.09.26.559538. bioRxiv. 2023. Update in: Genome Biol Evol. 2024 Jun 4;16(6):evae116. doi: 10.1093/gbe/evae116 PMID: 37873246 Free PMC article. Updated. Preprint.
-
The birth of a bacterial tRNA gene by large-scale, tandem duplication events.Elife. 2020 Oct 30;9:e57947. doi: 10.7554/eLife.57947. Elife. 2020. PMID: 33124983 Free PMC article.
-
Construction of anti-codon table of the plant kingdom and evolution of tRNA selenocysteine (tRNASec).BMC Genomics. 2020 Nov 19;21(1):804. doi: 10.1186/s12864-020-07216-3. BMC Genomics. 2020. PMID: 33213362 Free PMC article.
-
Large-scale duplication events underpin population-level flexibility in tRNA gene copy number in Pseudomonas fluorescens SBW25.Nucleic Acids Res. 2024 Mar 21;52(5):2446-2462. doi: 10.1093/nar/gkae049. Nucleic Acids Res. 2024. PMID: 38296823 Free PMC article.
-
Genome engineering on size reduction and complexity simplification: A review.J Adv Res. 2024 Jun;60:159-171. doi: 10.1016/j.jare.2023.07.006. Epub 2023 Jul 12. J Adv Res. 2024. PMID: 37442424 Free PMC article. Review.
References
-
- Ikemura T. Correlation between the abundance of Escherichia coli transfer RNAs and the occurrence of the respective codons in its protein genes: a proposal for a synonymous codon choice that is optimal for the E. coli translational system. J. Mol. Biol. 1981;151:389–409. - PubMed
Publication types
MeSH terms
Substances
LinkOut - more resources
Full Text Sources
Other Literature Sources
Miscellaneous