Human embryonic-stem-cell-derived cardiomyocytes regenerate non-human primate hearts
- PMID: 24776797
- PMCID: PMC4154594
- DOI: 10.1038/nature13233
Human embryonic-stem-cell-derived cardiomyocytes regenerate non-human primate hearts
Abstract
Pluripotent stem cells provide a potential solution to current epidemic rates of heart failure by providing human cardiomyocytes to support heart regeneration. Studies of human embryonic-stem-cell-derived cardiomyocytes (hESC-CMs) in small-animal models have shown favourable effects of this treatment. However, it remains unknown whether clinical-scale hESC-CM transplantation is feasible, safe or can provide sufficient myocardial regeneration. Here we show that hESC-CMs can be produced at a clinical scale (more than one billion cells per batch) and cryopreserved with good viability. Using a non-human primate model of myocardial ischaemia followed by reperfusion, we show that cryopreservation and intra-myocardial delivery of one billion hESC-CMs generates extensive remuscularization of the infarcted heart. The hESC-CMs showed progressive but incomplete maturation over a 3-month period. Grafts were perfused by host vasculature, and electromechanical junctions between graft and host myocytes were present within 2 weeks of engraftment. Importantly, grafts showed regular calcium transients that were synchronized to the host electrocardiogram, indicating electromechanical coupling. In contrast to small-animal models, non-fatal ventricular arrhythmias were observed in hESC-CM-engrafted primates. Thus, hESC-CMs can remuscularize substantial amounts of the infarcted monkey heart. Comparable remuscularization of a human heart should be possible, but potential arrhythmic complications need to be overcome.
Figures
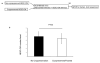
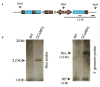
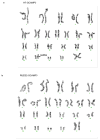
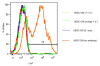
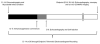
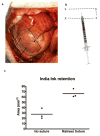
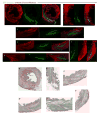
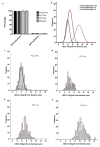
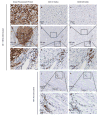
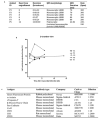
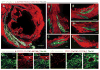
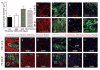
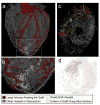
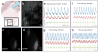
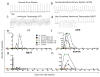
Comment in
-
Embryonic stem cell-derived cardiac myocytes are not ready for human trials.Circ Res. 2014 Jul 18;115(3):335-8. doi: 10.1161/CIRCRESAHA.114.304616. Epub 2014 Jun 16. Circ Res. 2014. PMID: 24935962 Free PMC article.
Similar articles
-
Human embryonic stem cell-derived cardiomyocytes restore function in infarcted hearts of non-human primates.Nat Biotechnol. 2018 Aug;36(7):597-605. doi: 10.1038/nbt.4162. Epub 2018 Jul 2. Nat Biotechnol. 2018. PMID: 29969440 Free PMC article.
-
Human ES-cell-derived cardiomyocytes electrically couple and suppress arrhythmias in injured hearts.Nature. 2012 Sep 13;489(7415):322-5. doi: 10.1038/nature11317. Nature. 2012. PMID: 22864415 Free PMC article.
-
The use of aggregates of purified cardiomyocytes derived from human ESCs for functional engraftment after myocardial infarction.Biomaterials. 2013 May;34(16):4013-4026. doi: 10.1016/j.biomaterials.2013.02.022. Epub 2013 Mar 5. Biomaterials. 2013. PMID: 23465823
-
Cardiac regeneration using pluripotent stem cells--progression to large animal models.Stem Cell Res. 2014 Nov;13(3 Pt B):654-65. doi: 10.1016/j.scr.2014.06.005. Epub 2014 Jul 6. Stem Cell Res. 2014. PMID: 25087896 Free PMC article. Review.
-
Stem Cell Banking and Its Impact on Cardiac Regenerative Medicine.Adv Exp Med Biol. 2016;951:163-178. doi: 10.1007/978-3-319-45457-3_14. Adv Exp Med Biol. 2016. PMID: 27837563 Review.
Cited by
-
SHOX2 overexpression favors differentiation of embryonic stem cells into cardiac pacemaker cells, improving biological pacing ability.Stem Cell Reports. 2015 Jan 13;4(1):129-142. doi: 10.1016/j.stemcr.2014.11.004. Epub 2014 Dec 18. Stem Cell Reports. 2015. PMID: 25533636 Free PMC article.
-
Cardiac engraftment of genetically-selected parthenogenetic stem cell-derived cardiomyocytes.PLoS One. 2015 Jun 25;10(6):e0131511. doi: 10.1371/journal.pone.0131511. eCollection 2015. PLoS One. 2015. PMID: 26110646 Free PMC article.
-
Proceedings: Moving Toward Cell-Based Therapies for Heart Disease.Stem Cells Transl Med. 2015 Aug;4(8):863-7. doi: 10.5966/sctm.2015-0118. Epub 2015 Jul 1. Stem Cells Transl Med. 2015. PMID: 26136501 Free PMC article.
-
Directed Differentiation of Zebrafish Pluripotent Embryonic Cells to Functional Cardiomyocytes.Stem Cell Reports. 2016 Sep 13;7(3):370-382. doi: 10.1016/j.stemcr.2016.07.020. Epub 2016 Aug 25. Stem Cell Reports. 2016. PMID: 27569061 Free PMC article.
-
OptoGap is an optogenetics-enabled assay for quantification of cell-cell coupling in multicellular cardiac tissue.Sci Rep. 2021 Apr 29;11(1):9310. doi: 10.1038/s41598-021-88573-1. Sci Rep. 2021. PMID: 33927252 Free PMC article.
References
Publication types
MeSH terms
Substances
Grants and funding
- P01 HL094374/HL/NHLBI NIH HHS/United States
- U01HL100405/HL/NHLBI NIH HHS/United States
- P01HL094374/HL/NHLBI NIH HHS/United States
- U01 HL100405/HL/NHLBI NIH HHS/United States
- P51 OD010425/OD/NIH HHS/United States
- R01 HL084642/HL/NHLBI NIH HHS/United States
- R01HL084642/HL/NHLBI NIH HHS/United States
- P01GM081619/GM/NIGMS NIH HHS/United States
- R01 HL117991/HL/NHLBI NIH HHS/United States
- P01 GM081619/GM/NIGMS NIH HHS/United States
- T32 GM007266/GM/NIGMS NIH HHS/United States
- P30 DK017047/DK/NIDDK NIH HHS/United States
LinkOut - more resources
Full Text Sources
Other Literature Sources
Medical
Research Materials