Dynamic localization of glucokinase and its regulatory protein in hypothalamic tanycytes
- PMID: 24739934
- PMCID: PMC3989220
- DOI: 10.1371/journal.pone.0094035
Dynamic localization of glucokinase and its regulatory protein in hypothalamic tanycytes
Abstract
Glucokinase (GK), the hexokinase involved in glucose sensing in pancreatic β cells, is also expressed in hypothalamic tanycytes, which cover the ventricular walls of the basal hypothalamus and are implicated in an indirect control of neuronal activity by glucose. Previously, we demonstrated that GK was preferentially localized in tanycyte nuclei in euglycemic rats, which has been reported in hepatocytes and is suggestive of the presence of the GK regulatory protein, GKRP. In the present study, GK intracellular localization in hypothalamic and hepatic tissues of the same rats under several glycemic conditions was compared using confocal microscopy and Western blot analysis. In the hypothalamus, increased GK nuclear localization was observed in hyperglycemic conditions; however, it was primarily localized in the cytoplasm in hepatic tissue under the same conditions. Both GK and GKRP were next cloned from primary cultures of tanycytes. Expression of GK by Escherichia coli revealed a functional cooperative protein with a S0.5 of 10 mM. GKRP, expressed in Saccharomyces cerevisiae, inhibited GK activity in vitro with a Ki 0.2 µM. We also demonstrated increased nuclear reactivity of both GK and GKRP in response to high glucose concentrations in tanycyte cultures. These data were confirmed using Western blot analysis of nuclear extracts. Results indicate that GK undergoes short-term regulation by nuclear compartmentalization. Thus, in tanycytes, GK can act as a molecular switch to arrest cellular responses to increased glucose.
Conflict of interest statement
Figures
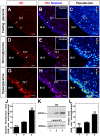
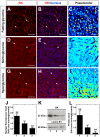
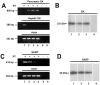
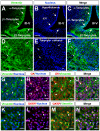
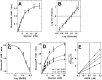
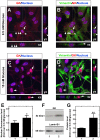
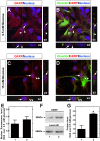
Similar articles
-
The role of tanycytes in hypothalamic glucosensing.J Cell Mol Med. 2015 Jul;19(7):1471-82. doi: 10.1111/jcmm.12590. Epub 2015 Jun 17. J Cell Mol Med. 2015. PMID: 26081217 Free PMC article. Review.
-
When a Little Bit More Makes the Difference: Expression Levels of GKRP Determines the Subcellular Localization of GK in Tanycytes.Front Neurosci. 2019 Mar 29;13:275. doi: 10.3389/fnins.2019.00275. eCollection 2019. Front Neurosci. 2019. PMID: 30983961 Free PMC article.
-
Glial glucokinase expression in adult and post-natal development of the hypothalamic region.ASN Neuro. 2010 May 25;2(3):e00035. doi: 10.1042/AN20090059. ASN Neuro. 2010. PMID: 20531973 Free PMC article.
-
GKRP-dependent modulation of feeding behavior by tanycyte-released monocarboxylates.Theranostics. 2022 Jan 3;12(4):1518-1536. doi: 10.7150/thno.66634. eCollection 2022. Theranostics. 2022. PMID: 35198055 Free PMC article.
-
Recent Updates on Glucokinase Activators and Glucokinase Regulatory Protein Disrupters for the Treatment of Type 2 Diabetes Mellitus.Curr Diabetes Rev. 2019;15(3):205-212. doi: 10.2174/1573399814666180724100749. Curr Diabetes Rev. 2019. PMID: 30039763 Review.
Cited by
-
Nutrient Sensing by Hypothalamic Tanycytes.Front Endocrinol (Lausanne). 2019 Apr 16;10:244. doi: 10.3389/fendo.2019.00244. eCollection 2019. Front Endocrinol (Lausanne). 2019. PMID: 31040827 Free PMC article. Review.
-
Insights into the role of neuronal glucokinase.Am J Physiol Endocrinol Metab. 2016 Jul 1;311(1):E42-55. doi: 10.1152/ajpendo.00034.2016. Epub 2016 May 17. Am J Physiol Endocrinol Metab. 2016. PMID: 27189932 Free PMC article. Review.
-
Connexin-43 Gap Junctions Are Responsible for the Hypothalamic Tanycyte-Coupled Network.Front Cell Neurosci. 2018 Nov 26;12:406. doi: 10.3389/fncel.2018.00406. eCollection 2018. Front Cell Neurosci. 2018. PMID: 30534054 Free PMC article.
-
Lactate activates hypothalamic POMC neurons by intercellular signaling.Sci Rep. 2021 Nov 4;11(1):21644. doi: 10.1038/s41598-021-00947-7. Sci Rep. 2021. PMID: 34737351 Free PMC article.
-
The role of tanycytes in hypothalamic glucosensing.J Cell Mol Med. 2015 Jul;19(7):1471-82. doi: 10.1111/jcmm.12590. Epub 2015 Jun 17. J Cell Mol Med. 2015. PMID: 26081217 Free PMC article. Review.
References
-
- Schwartz MW, Woods SC, Porte D Jr, Seeley RJ, Baskin DG (2000) Central nervous system control of food intake. Nature 404: 661–671. - PubMed
-
- Song Z, Routh VH (2005) Differential effects of glucose and lactate on glucosensing neurons in the ventromedial hypothalamic nucleus. Diabetes 54: 15–22. - PubMed
-
- Yang XJ, Kow LM, Funabashi T, Mobbs CV (1999) Hypothalamic glucose sensor: similarities to and differences from pancreatic beta-cell mechanisms. Diabetes 48: 1763–1772. - PubMed
-
- Chauvet N, Parmentier ML, Alonso G (1995) Transected axons of adult hypothalamo-neurohypophysial neurons regenerate along tanycytic processes. J Neurosci Res 41: 129–144. - PubMed
-
- Flament-Durand J, Brion JP (1985) Tanycytes: morphology and functions: a review. Int Rev Cytol 96: 121–155. - PubMed
Publication types
MeSH terms
Substances
Grants and funding
LinkOut - more resources
Full Text Sources
Other Literature Sources