Dynamic regulation of macroautophagy by distinctive ubiquitin-like proteins
- PMID: 24699082
- PMCID: PMC4036234
- DOI: 10.1038/nsmb.2787
Dynamic regulation of macroautophagy by distinctive ubiquitin-like proteins
Abstract
Autophagy complements the ubiquitin-proteasome system in mediating protein turnover. Whereas the proteasome degrades individual proteins modified with ubiquitin chains, autophagy degrades many proteins and organelles en masse. Macromolecules destined for autophagic degradation are 'selected' through sequestration within a specialized double-membrane compartment termed the phagophore, the precursor to an autophagosome, and then are hydrolyzed in a lysosome- or vacuole-dependent manner. Notably, a pair of distinctive ubiquitin-like proteins (UBLs), Atg8 and Atg12, regulate degradation by autophagy in unique ways by controlling autophagosome biogenesis and recruitment of specific cargos during selective autophagy. Here we review structural mechanisms underlying the functions and conjugation of these UBLs that are specialized to provide interaction platforms linked to phagophore membranes.
Figures
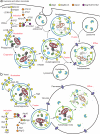
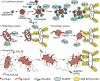
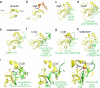
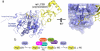
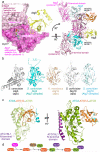
Similar articles
-
Interactions between autophagy receptors and ubiquitin-like proteins form the molecular basis for selective autophagy.Mol Cell. 2014 Jan 23;53(2):167-78. doi: 10.1016/j.molcel.2013.12.014. Mol Cell. 2014. PMID: 24462201 Review.
-
In vitro systems for Atg8 lipidation.Methods. 2015 Mar;75:37-43. doi: 10.1016/j.ymeth.2014.11.004. Epub 2014 Nov 13. Methods. 2015. PMID: 25461810 Free PMC article.
-
In vitro assays of lipidation of Mammalian Atg8 homologs.Curr Protoc Cell Biol. 2014 Sep 2;64:11.20.1-13. doi: 10.1002/0471143030.cb1120s64. Curr Protoc Cell Biol. 2014. PMID: 25181299 Review.
-
The Roles of Ubiquitin in Mediating Autophagy.Cells. 2020 Sep 2;9(9):2025. doi: 10.3390/cells9092025. Cells. 2020. PMID: 32887506 Free PMC article. Review.
-
Autophagy, proteases and the sense of balance.Autophagy. 2010 Oct;6(7):961-3. doi: 10.4161/auto.6.7.13065. Epub 2010 Oct 19. Autophagy. 2010. PMID: 20724821
Cited by
-
Evaluating the Mode of Antifungal Action of Heat-Stable Antifungal Factor (HSAF) in Neurospora crassa.J Fungi (Basel). 2022 Mar 1;8(3):252. doi: 10.3390/jof8030252. J Fungi (Basel). 2022. PMID: 35330254 Free PMC article.
-
NBR1 is involved in selective pexophagy in filamentous ascomycetes and can be functionally replaced by a tagged version of its human homolog.Autophagy. 2019 Jan;15(1):78-97. doi: 10.1080/15548627.2018.1507440. Epub 2018 Sep 6. Autophagy. 2019. PMID: 30081713 Free PMC article.
-
The C-terminal region of ATG101 bridges ULK1 and PtdIns3K complex in autophagy initiation.Autophagy. 2018;14(12):2104-2116. doi: 10.1080/15548627.2018.1504716. Epub 2018 Aug 16. Autophagy. 2018. PMID: 30081750 Free PMC article.
-
IL-36α and Lipopolysaccharide Cooperatively Induce Autophagy by Triggering Pro-Autophagic Biased Signaling.Biomedicines. 2021 Oct 26;9(11):1541. doi: 10.3390/biomedicines9111541. Biomedicines. 2021. PMID: 34829770 Free PMC article.
-
Glial Cell Line-Derived Neurotrophic Factor Enhances Autophagic Flux in Mouse and Rat Hepatocytes and Protects Against Palmitate Lipotoxicity.Hepatology. 2019 Jun;69(6):2455-2470. doi: 10.1002/hep.30541. Epub 2019 Mar 22. Hepatology. 2019. PMID: 30715741 Free PMC article.
References
-
- Oku M, Sakai Y. Peroxisomes as dynamic organelles: autophagic degradation. Febs J. 2010;277:3289–3294. doi:10.1111/j.1742-4658.2010.07741.x. - PubMed
Publication types
MeSH terms
Substances
Grants and funding
LinkOut - more resources
Full Text Sources
Other Literature Sources
Research Materials