A cortical circuit for gain control by behavioral state
- PMID: 24630718
- PMCID: PMC4041382
- DOI: 10.1016/j.cell.2014.01.050
A cortical circuit for gain control by behavioral state
Abstract
The brain's response to sensory input is strikingly modulated by behavioral state. Notably, the visual response of mouse primary visual cortex (V1) is enhanced by locomotion, a tractable and accessible example of a time-locked change in cortical state. The neural circuits that transmit behavioral state to sensory cortex to produce this modulation are unknown. In vivo calcium imaging of behaving animals revealed that locomotion activates vasoactive intestinal peptide (VIP)-positive neurons in mouse V1 independent of visual stimulation and largely through nicotinic inputs from basal forebrain. Optogenetic activation of VIP neurons increased V1 visual responses in stationary awake mice, artificially mimicking the effect of locomotion, and photolytic damage of VIP neurons abolished the enhancement of V1 responses by locomotion. These findings establish a cortical circuit for the enhancement of visual response by locomotion and provide a potential common circuit for the modulation of sensory processing by behavioral state.
Copyright © 2014 Elsevier Inc. All rights reserved.
Figures
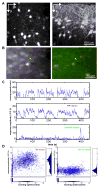
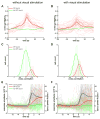
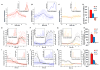
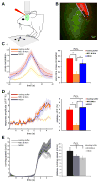
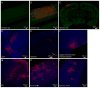
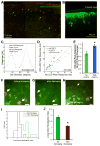
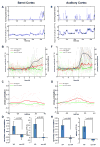
Comment in
-
Visual circuits get the VIP treatment.Cell. 2014 Mar 13;156(6):1123-1124. doi: 10.1016/j.cell.2014.02.043. Cell. 2014. PMID: 24630713
Similar articles
-
Behavioral-state modulation of inhibition is context-dependent and cell type specific in mouse visual cortex.Elife. 2016 Aug 23;5:e14985. doi: 10.7554/eLife.14985. Elife. 2016. PMID: 27552056 Free PMC article.
-
Visual processing mode switching regulated by VIP cells.Sci Rep. 2017 May 12;7(1):1843. doi: 10.1038/s41598-017-01830-0. Sci Rep. 2017. PMID: 28500299 Free PMC article.
-
Effects of Locomotion in Auditory Cortex Are Not Mediated by the VIP Network.Front Neural Circuits. 2021 Apr 7;15:618881. doi: 10.3389/fncir.2021.618881. eCollection 2021. Front Neural Circuits. 2021. PMID: 33897378 Free PMC article.
-
Stimulus-specific enhancement in mouse visual cortex requires GABA but not VIP-peptide release from VIP interneurons.J Neurophysiol. 2024 Jul 1;132(1):34-44. doi: 10.1152/jn.00463.2023. Epub 2024 May 22. J Neurophysiol. 2024. PMID: 38774975 Free PMC article.
-
A Neural Circuit That Controls Cortical State, Plasticity, and the Gain of Sensory Responses in Mouse.Cold Spring Harb Symp Quant Biol. 2014;79:1-9. doi: 10.1101/sqb.2014.79.024927. Epub 2015 May 6. Cold Spring Harb Symp Quant Biol. 2014. PMID: 25948638 Free PMC article. Review.
Cited by
-
Gamma rhythms and visual information in mouse V1 specifically modulated by somatostatin+ neurons in reticular thalamus.Elife. 2021 Apr 12;10:e61437. doi: 10.7554/eLife.61437. Elife. 2021. PMID: 33843585 Free PMC article.
-
A systematic review and Bayesian meta-analysis provide evidence for an effect of acute physical activity on cognition in young adults.Commun Psychol. 2024 Aug 28;2(1):82. doi: 10.1038/s44271-024-00124-2. Commun Psychol. 2024. PMID: 39242965 Free PMC article.
-
Changing the Cortical Conductor's Tempo: Neuromodulation of the Claustrum.Front Neural Circuits. 2021 May 13;15:658228. doi: 10.3389/fncir.2021.658228. eCollection 2021. Front Neural Circuits. 2021. PMID: 34054437 Free PMC article. Review.
-
Hierarchy in sensory processing reflected by innervation balance on cortical interneurons.Sci Adv. 2021 May 14;7(20):eabf5676. doi: 10.1126/sciadv.abf5676. Print 2021 May. Sci Adv. 2021. PMID: 33990327 Free PMC article.
-
Performance in a GO/NOGO perceptual task reflects a balance between impulsive and instrumental components of behaviour.Sci Rep. 2016 Jun 7;6:27389. doi: 10.1038/srep27389. Sci Rep. 2016. PMID: 27272438 Free PMC article.
References
Publication types
MeSH terms
Substances
Grants and funding
LinkOut - more resources
Full Text Sources
Other Literature Sources
Molecular Biology Databases