Quality control of PSII: behavior of PSII in the highly crowded grana thylakoids under excessive light
- PMID: 24610582
- PMCID: PMC4080270
- DOI: 10.1093/pcp/pcu043
Quality control of PSII: behavior of PSII in the highly crowded grana thylakoids under excessive light
Abstract
The grana thylakoids of higher plant chloroplasts are crowded with PSII and the associated light-harvesting complexes (LHCIIs). They constitute supercomplexes, and often form semi-crystalline arrays in the grana. The crowded condition of the grana may be necessary for efficient trapping of excitation energy by LHCII under weak light, but it might hinder proper movement of LHCII necessary for reversible aggregation of LHCII in the energy-dependent quenching of Chl fluorescence under moderate high light. When the thylakoids are illuminated with extreme high light, the reaction center-binding D1 protein of PSII is photodamaged, and the damaged protein migrates to the grana margins for degradation and subsequent repair. In both moderate and extreme high-light conditions, fluidity of the thylakoid membrane is crucial. In this review, we first provide an overview of photoprotective processes, then discuss changes in membrane fluidity and mobility of the protein complexes in the grana under excessive light, which are closely associated with photoprotection of PSII. We hypothesize that reversible aggregation of LHCII, which is necessary to avoid light stress under moderate high light, and swift turnover of the photodamaged D1 protein under extreme high light are threatened by irreversible protein aggregation induced by reactive oxygen species in photochemical reactions.
Keywords: Light stress; Membrane crowdedness; Non-photochemical quenching; Photoinhibition; Photosystem II; Protein aggregation.
© The Author 2014. Published by Oxford University Press on behalf of Japanese Society of Plant Physiologists.
Figures
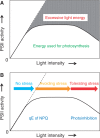
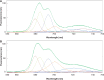
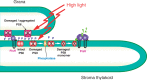
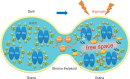
Similar articles
-
Close Relationships Between the PSII Repair Cycle and Thylakoid Membrane Dynamics.Plant Cell Physiol. 2016 Jun;57(6):1115-22. doi: 10.1093/pcp/pcw050. Epub 2016 Mar 26. Plant Cell Physiol. 2016. PMID: 27017619 Review.
-
Effect of light on the rearrangements of PSI super-and megacomplexes in the non-appressed thylakoid domains of maize mesophyll chloroplasts.Plant Sci. 2020 Dec;301:110655. doi: 10.1016/j.plantsci.2020.110655. Epub 2020 Sep 6. Plant Sci. 2020. PMID: 33218624
-
How paired PSII-LHCII supercomplexes mediate the stacking of plant thylakoid membranes unveiled by structural mass-spectrometry.Nat Commun. 2020 Mar 13;11(1):1361. doi: 10.1038/s41467-020-15184-1. Nat Commun. 2020. PMID: 32170184 Free PMC article.
-
Monomeric light harvesting complexes enhance excitation energy transfer from LHCII to PSII and control their lateral spacing in thylakoids.Biochim Biophys Acta Bioenerg. 2020 Apr 1;1861(4):148035. doi: 10.1016/j.bbabio.2019.06.007. Epub 2019 Jun 19. Biochim Biophys Acta Bioenerg. 2020. PMID: 31226317
-
Dynamics of photosystem II: a proteomic approach to thylakoid protein complexes.J Exp Bot. 2005 Jan;56(411):347-56. doi: 10.1093/jxb/eri041. Epub 2004 Nov 29. J Exp Bot. 2005. PMID: 15569703 Review.
Cited by
-
Structural Entities Associated with Different Lipid Phases of Plant Thylakoid Membranes-Selective Susceptibilities to Different Lipases and Proteases.Cells. 2022 Aug 28;11(17):2681. doi: 10.3390/cells11172681. Cells. 2022. PMID: 36078087 Free PMC article.
-
Fine-tuning the photosynthetic light harvesting apparatus for improved photosynthetic efficiency and biomass yield.Sci Rep. 2019 Sep 10;9(1):13028. doi: 10.1038/s41598-019-49545-8. Sci Rep. 2019. PMID: 31506512 Free PMC article.
-
An Overview of Biomembrane Functions in Plant Responses to High-Temperature Stress.Front Plant Sci. 2018 Jul 3;9:915. doi: 10.3389/fpls.2018.00915. eCollection 2018. Front Plant Sci. 2018. PMID: 30018629 Free PMC article. Review.
-
Quality Control of Photosystem II: The Mechanisms for Avoidance and Tolerance of Light and Heat Stresses are Closely Linked to Membrane Fluidity of the Thylakoids.Front Plant Sci. 2016 Aug 2;7:1136. doi: 10.3389/fpls.2016.01136. eCollection 2016. Front Plant Sci. 2016. PMID: 27532009 Free PMC article. Review.
-
Chlorophyll fluorescence analysis revealed essential roles of FtsH11 protease in regulation of the adaptive responses of photosynthetic systems to high temperature.BMC Plant Biol. 2018 Jan 10;18(1):11. doi: 10.1186/s12870-018-1228-2. BMC Plant Biol. 2018. PMID: 29320985 Free PMC article.
References
-
- Albertsson P. A quantitative model of the domain structure of the photosynthetic membrane. Trends Plant Sci. 2001;6:349–358. - PubMed
-
- Allen JF. Protein phosphorylation in regulation of photosynthesis. Biochim. Biophys. Acta. 1992;1098:275–335. - PubMed
-
- Allen JF, Forsberg J. Molecular recognition in thylakoid structure and function. Trends Plant Sci. 2001;6:317–326. - PubMed
-
- Anderson JM. Insights into the consequences of grana stacking of thylakoid membranes in vascular plants: a personal perspective. Aust. J. Plant Physiol. 1999;26:625–639.
Publication types
MeSH terms
Substances
LinkOut - more resources
Full Text Sources
Other Literature Sources