Modelling of human low frequency sound localization acuity demonstrates dominance of spatial variation of interaural time difference and suggests uniform just-noticeable differences in interaural time difference
- PMID: 24558468
- PMCID: PMC3928360
- DOI: 10.1371/journal.pone.0089033
Modelling of human low frequency sound localization acuity demonstrates dominance of spatial variation of interaural time difference and suggests uniform just-noticeable differences in interaural time difference
Abstract
Sound source localization is critical to animal survival and for identification of auditory objects. We investigated the acuity with which humans localize low frequency, pure tone sounds using timing differences between the ears. These small differences in time, known as interaural time differences or ITDs, are identified in a manner that allows localization acuity of around 1° at the midline. Acuity, a relative measure of localization ability, displays a non-linear variation as sound sources are positioned more laterally. All species studied localize sounds best at the midline and progressively worse as the sound is located out towards the side. To understand why sound localization displays this variation with azimuthal angle, we took a first-principles, systemic, analytical approach to model localization acuity. We calculated how ITDs vary with sound frequency, head size and sound source location for humans. This allowed us to model ITD variation for previously published experimental acuity data and determine the distribution of just-noticeable differences in ITD. Our results suggest that the best-fit model is one whereby just-noticeable differences in ITDs are identified with uniform or close to uniform sensitivity across the physiological range. We discuss how our results have several implications for neural ITD processing in different species as well as development of the auditory system.
Conflict of interest statement
Figures
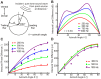
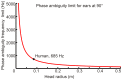
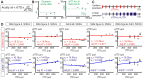
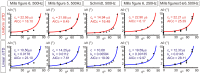
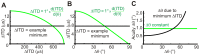
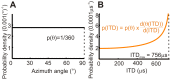
Similar articles
-
A common periodic representation of interaural time differences in mammalian cortex.Neuroimage. 2018 Feb 15;167:95-103. doi: 10.1016/j.neuroimage.2017.11.012. Epub 2017 Nov 7. Neuroimage. 2018. PMID: 29122721 Free PMC article.
-
Localization of broadband sounds carrying interaural time differences: Effects of frequency, reference location, and interaural coherence.J Acoust Soc Am. 2018 Oct;144(4):2225. doi: 10.1121/1.5058776. J Acoust Soc Am. 2018. PMID: 30404466
-
Combination of Interaural Level and Time Difference in Azimuthal Sound Localization in Owls.eNeuro. 2017 Dec 14;4(6):ENEURO.0238-17.2017. doi: 10.1523/ENEURO.0238-17.2017. eCollection 2017 Nov-Dec. eNeuro. 2017. PMID: 29379866 Free PMC article.
-
Auditory processing of interaural timing information: new insights.J Neurosci Res. 2001 Dec 15;66(6):1035-46. doi: 10.1002/jnr.10103. J Neurosci Res. 2001. PMID: 11746435 Review.
-
The modulation by intensity of the processing of interaural timing cues for localizing sounds.Mol Neurobiol. 2009 Oct;40(2):157-65. doi: 10.1007/s12035-009-8078-8. Epub 2009 Jul 11. Mol Neurobiol. 2009. PMID: 19593674 Review.
Cited by
-
An exploration of spatial auditory BCI paradigms with different sounds: music notes versus beeps.Cogn Neurodyn. 2016 Jun;10(3):201-9. doi: 10.1007/s11571-016-9377-1. Epub 2016 Jan 23. Cogn Neurodyn. 2016. PMID: 27275376 Free PMC article.
-
Detection of Spatially Localized Sounds Is Robust to Saccades and Concurrent Eye Movement-Related Eardrum Oscillations (EMREOs).J Neurosci. 2023 Nov 8;43(45):7668-7677. doi: 10.1523/JNEUROSCI.0818-23.2023. Epub 2023 Sep 21. J Neurosci. 2023. PMID: 37734948 Free PMC article.
-
Subcortical pathways: Towards a better understanding of auditory disorders.Hear Res. 2018 May;362:48-60. doi: 10.1016/j.heares.2018.01.008. Epub 2018 Jan 31. Hear Res. 2018. PMID: 29395615 Free PMC article. Review.
-
Spatial variation in signal and sensory precision both constrain auditory acuity at high frequencies.Hear Res. 2018 Dec;370:65-73. doi: 10.1016/j.heares.2018.10.002. Epub 2018 Oct 4. Hear Res. 2018. PMID: 30326382 Free PMC article.
-
Binaural-cue Weighting and Training-Induced Reweighting Across Frequencies.Trends Hear. 2022 Jan-Dec;26:23312165221104872. doi: 10.1177/23312165221104872. Trends Hear. 2022. PMID: 35791626 Free PMC article.
References
-
- Rayleigh L (1876) Our perception of the direction of a source of sound. Nature 14: 32–33.
-
- Stevens SS, Newman EB (1936) The localisation of actual sources of sound. Am J Psychol 48: 297–306.
-
- Sandel T, Teas D (1955) Localization of Sound from Single and Paired Sources. J Acoust Soc Am 27: 842–852.
Publication types
MeSH terms
Grants and funding
LinkOut - more resources
Full Text Sources
Other Literature Sources