Hijacking of RIG-I signaling proteins into virus-induced cytoplasmic structures correlates with the inhibition of type I interferon responses
- PMID: 24478431
- PMCID: PMC3993744
- DOI: 10.1128/JVI.03021-13
Hijacking of RIG-I signaling proteins into virus-induced cytoplasmic structures correlates with the inhibition of type I interferon responses
Abstract
Recognition of viral pathogens by the retinoic acid-inducible gene I (RIG-I)-like receptor (RLR) family results in the activation of type I interferon (IFN) responses. To avoid this response, most viruses have evolved strategies that target different essential steps in the activation of host innate immunity. In this study, we report that the nonstructural protein NSs of the newly described severe fever with thrombocytopenia syndrome virus (SFTSV) is a potent inhibitor of IFN responses. The SFTSV NSs protein was found to inhibit the activation of the beta interferon (IFN-β) promoter induced by viral infection and by a RIG-I ligand. Astonishingly, we found that SFTSV NSs interacts with and relocalizes RIG-I, the E3 ubiquitin ligase TRIM25, and TANK-binding kinase 1 (TBK1) into SFTSV NSs-induced cytoplasmic structures. Interestingly, formation of these SFTSV NSs-induced structures occurred in the absence of the Atg7 gene, a gene essential for autophagy. Furthermore, confocal microscopy studies revealed that these SFTSV NSs-induced structures colocalize with Rab5 but not with Golgi apparatus or endoplasmic reticulum markers. Altogether, the data suggest that sequestration of RIG-I signaling molecules into endosome-like structures may be the mechanism used by SFTSV to inhibit IFN responses and point toward a novel mechanism for the suppression of IFN responses.
Importance: The mechanism by which the newly described SFTSV inhibits host antiviral responses has not yet been fully characterized. In this study, we describe the redistribution of RIG-I signaling components into virus-induced cytoplasmic structures in cells infected with SFTSV. This redistribution correlates with the inhibition of host antiviral responses. Further characterization of the interplay between the viral protein and components of the IFN responses could potentially provide targets for the rational development of therapeutic interventions.
Figures
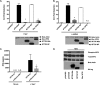
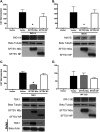
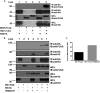
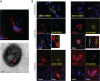
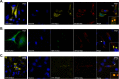
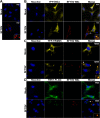
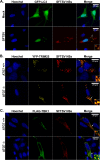
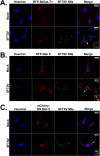
Similar articles
-
A RIG-I-like receptor directs antiviral responses to a bunyavirus and is antagonized by virus-induced blockade of TRIM25-mediated ubiquitination.J Biol Chem. 2020 Jul 10;295(28):9691-9711. doi: 10.1074/jbc.RA120.013973. Epub 2020 May 29. J Biol Chem. 2020. PMID: 32471869 Free PMC article.
-
Two Conserved Amino Acids within the NSs of Severe Fever with Thrombocytopenia Syndrome Phlebovirus Are Essential for Anti-interferon Activity.J Virol. 2018 Sep 12;92(19):e00706-18. doi: 10.1128/JVI.00706-18. Print 2018 Oct 1. J Virol. 2018. PMID: 30021900 Free PMC article.
-
Nonstructural Protein of Severe Fever with Thrombocytopenia Syndrome Phlebovirus Inhibits TBK1 to Evade Interferon-Mediated Response.J Microbiol Biotechnol. 2021 Feb 28;31(2):226-232. doi: 10.4014/jmb.2008.08048. J Microbiol Biotechnol. 2021. PMID: 33397830 Free PMC article.
-
Unraveling the Underlying Interaction Mechanism Between Dabie bandavirus and Innate Immune Response.Front Immunol. 2021 May 27;12:676861. doi: 10.3389/fimmu.2021.676861. eCollection 2021. Front Immunol. 2021. PMID: 34122440 Free PMC article. Review.
-
Phleboviruses and the Type I Interferon Response.Viruses. 2016 Jun 22;8(6):174. doi: 10.3390/v8060174. Viruses. 2016. PMID: 27338447 Free PMC article. Review.
Cited by
-
Overview of the immunological mechanism underlying severe fever with thrombocytopenia syndrome (Review).Int J Mol Med. 2022 Sep;50(3):118. doi: 10.3892/ijmm.2022.5174. Epub 2022 Jul 20. Int J Mol Med. 2022. PMID: 35856413 Free PMC article. Review.
-
SAFA initiates innate immunity against cytoplasmic RNA virus SFTSV infection.PLoS Pathog. 2021 Nov 17;17(11):e1010070. doi: 10.1371/journal.ppat.1010070. eCollection 2021 Nov. PLoS Pathog. 2021. PMID: 34788350 Free PMC article.
-
Correlation of cytokine level with the severity of severe fever with thrombocytopenia syndrome.Virol J. 2017 Jan 13;14(1):6. doi: 10.1186/s12985-016-0677-1. Virol J. 2017. PMID: 28086978 Free PMC article.
-
Negri bodies are viral factories with properties of liquid organelles.Nat Commun. 2017 Jul 5;8(1):58. doi: 10.1038/s41467-017-00102-9. Nat Commun. 2017. PMID: 28680096 Free PMC article.
-
The Roles of TRIMs in Antiviral Innate Immune Signaling.Front Cell Infect Microbiol. 2021 Mar 15;11:628275. doi: 10.3389/fcimb.2021.628275. eCollection 2021. Front Cell Infect Microbiol. 2021. PMID: 33791238 Free PMC article. Review.
References
Publication types
MeSH terms
Substances
Grants and funding
- T32 AI007647/AI/NIAID NIH HHS/United States
- CA125123/CA/NCI NIH HHS/United States
- U54 AI057156/AI/NIAID NIH HHS/United States
- T32 AI007536/AI/NIAID NIH HHS/United States
- AI007647/AI/NIAID NIH HHS/United States
- UC7 AI094660/AI/NIAID NIH HHS/United States
- HHSN272201000040I/AI/NIAID NIH HHS/United States
- P30 AI036211/AI/NIAID NIH HHS/United States
- S10 RR024574/RR/NCRR NIH HHS/United States
- RR024574/RR/NCRR NIH HHS/United States
- HHSN27200004/D04/PHS HHS/United States
- AI036211/AI/NIAID NIH HHS/United States
- U19 AI083025/AI/NIAID NIH HHS/United States
- P30 CA125123/CA/NCI NIH HHS/United States
- AI007536/AI/NIAID NIH HHS/United States
- U54AI057156/AI/NIAID NIH HHS/United States
LinkOut - more resources
Full Text Sources
Other Literature Sources
Molecular Biology Databases
Research Materials
Miscellaneous