Chondrocyte β-catenin signaling regulates postnatal bone remodeling through modulation of osteoclast formation in a murine model
- PMID: 24431282
- PMCID: PMC3932359
- DOI: 10.1002/art.38195
Chondrocyte β-catenin signaling regulates postnatal bone remodeling through modulation of osteoclast formation in a murine model
Erratum in
-
Incorrect Figure in the Article by Wang et al (Arthritis Rheumatol, January 2014).Arthritis Rheumatol. 2015 Sep;67(9):2465. doi: 10.1002/art.39327. Arthritis Rheumatol. 2015. PMID: 26309052 No abstract available.
Abstract
Objective: To investigate whether β-catenin signaling in chondrocytes regulates osteoclastogenesis, thereby contributing to postnatal bone growth and bone remodeling.
Methods: Mice with conditional knockout (cKO) or conditional activation (cAct) of chondrocyte-specific β-catenin were generated. Changes in bone mass, osteoclast numbers, and osteoblast activity were examined. The mechanisms by which β-catenin signaling in chondrocytes regulates osteoclast formation were determined.
Results: The β-catenin cKO mice developed localized bone loss, whereas cAct mice developed a high bone mass phenotype. Histologic findings suggested that these phenotypes were caused primarily by impaired osteoclast formation, rather than impaired bone formation. Further molecular signaling analyses revealed that β-catenin signaling controlled this process by regulating the expression of the RANKL and osteoprotegerin (OPG) genes in chondrocytes. Activation of β-catenin signaling in chondrocytes suppressed Rankl gene transcription through a glucocorticoid receptor-dependent mechanism. The severe bone loss phenotype observed in β-catenin cKO mice was largely restored by treatment with human recombinant OPG or transgenic overexpression of Opg in chondrocytes.
Conclusion: β-catenin signaling in chondrocytes plays a key role in postnatal bone growth and bone remodeling through its regulation of osteoclast formation.
Copyright © 2014 by the American College of Rheumatology.
Figures
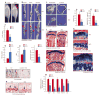
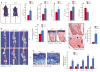
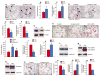
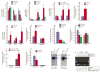
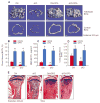
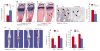
Similar articles
-
Chondrocyte FGFR3 Regulates Bone Mass by Inhibiting Osteogenesis.J Biol Chem. 2016 Nov 25;291(48):24912-24921. doi: 10.1074/jbc.M116.730093. Epub 2016 Oct 11. J Biol Chem. 2016. PMID: 27729453 Free PMC article.
-
Regulatory mechanisms of RANKL presentation to osteoclast precursors.Curr Osteoporos Rep. 2014 Mar;12(1):115-20. doi: 10.1007/s11914-014-0189-0. Curr Osteoporos Rep. 2014. PMID: 24477414 Review.
-
Activation of mTORC1 in B Lymphocytes Promotes Osteoclast Formation via Regulation of β-Catenin and RANKL/OPG.J Bone Miner Res. 2016 Jul;31(7):1320-33. doi: 10.1002/jbmr.2800. Epub 2016 Mar 4. J Bone Miner Res. 2016. PMID: 26825871
-
Naringin increases osteoprotegerin expression in fibroblasts from periprosthetic membrane by the Wnt/β-catenin signaling pathway.J Orthop Surg Res. 2020 Dec 10;15(1):600. doi: 10.1186/s13018-020-02145-z. J Orthop Surg Res. 2020. PMID: 33302980 Free PMC article.
-
Regulatory mechanisms of osteoblast and osteoclast differentiation.Oral Dis. 2002 May;8(3):147-59. doi: 10.1034/j.1601-0825.2002.01829.x. Oral Dis. 2002. PMID: 12108759 Review.
Cited by
-
Subchondral bone microenvironment in osteoarthritis and pain.Bone Res. 2021 Mar 17;9(1):20. doi: 10.1038/s41413-021-00147-z. Bone Res. 2021. PMID: 33731688 Free PMC article. Review.
-
Hippuric acid and 3-(3-hydroxyphenyl) propionic acid inhibit murine osteoclastogenesis through RANKL-RANK independent pathway.J Cell Physiol. 2020 Jan;235(1):599-610. doi: 10.1002/jcp.28998. Epub 2019 Jul 4. J Cell Physiol. 2020. PMID: 31271661 Free PMC article.
-
Reducing dietary loading decreases mouse temporomandibular joint degradation induced by anterior crossbite prosthesis.Osteoarthritis Cartilage. 2014 Feb;22(2):302-12. doi: 10.1016/j.joca.2013.11.014. Epub 2013 Dec 5. Osteoarthritis Cartilage. 2014. PMID: 24316289 Free PMC article.
-
Vital Roles of β-catenin in Trans-differentiation of Chondrocytes to Bone Cells.Int J Biol Sci. 2018 Jan 1;14(1):1-9. doi: 10.7150/ijbs.23165. eCollection 2018. Int J Biol Sci. 2018. PMID: 29483820 Free PMC article.
-
Shen (Kidney)-tonifying principle for primary osteoporosis: to treat both the disease and the Chinese medicine syndrome.Chin J Integr Med. 2015 Sep;21(9):656-61. doi: 10.1007/s11655-015-2306-z. Epub 2015 Oct 3. Chin J Integr Med. 2015. PMID: 26432789 Review.
References
-
- Adams CS, Shapiro IM. The fate of the terminally differentiated chondrocyte: evidence for microenvironmental regulation of chondrocyte apoptosis. Crit Rev Oral Biol Med. 2002;13:465–73. - PubMed
-
- Gibson G. Active role of chondrocyte apoptosis in endochondral ossification. Microsc Res Tech. 1998;43:191–204. - PubMed
Publication types
MeSH terms
Substances
Grants and funding
LinkOut - more resources
Full Text Sources
Other Literature Sources