How sticky should a virus be? The impact of virus binding and release on transmission fitness using influenza as an example
- PMID: 24430126
- PMCID: PMC3899878
- DOI: 10.1098/rsif.2013.1083
How sticky should a virus be? The impact of virus binding and release on transmission fitness using influenza as an example
Abstract
Budding viruses face a trade-off: virions need to efficiently attach to and enter uninfected cells while newly generated virions need to efficiently detach from infected cells. The right balance between attachment and detachment-the right amount of stickiness-is needed for maximum fitness. Here, we design and analyse a mathematical model to study in detail the impact of attachment and detachment rates on virus fitness. We apply our model to influenza, where stickiness is determined by a balance of the haemagglutinin (HA) and neuraminidase (NA) proteins. We investigate how drugs, the adaptive immune response and vaccines impact influenza stickiness and fitness. Our model suggests that the location in the 'stickiness landscape' of the virus determines how well interventions such as drugs or vaccines are expected to work. We discuss why hypothetical NA enhancer drugs might occasionally perform better than the currently available NA inhibitors in reducing virus fitness. We show that an increased antibody or T-cell-mediated immune response leads to maximum fitness at higher stickiness. We further show that antibody-based vaccines targeting mainly HA or NA, which leads to a shift in stickiness, might reduce virus fitness above what can be achieved by the direct immunological action of the vaccine. Overall, our findings provide potentially useful conceptual insights for future vaccine and drug development and can be applied to other budding viruses beyond influenza.
Keywords: drug treatment; influenza; vaccines; within-host model.
Figures
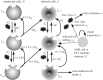





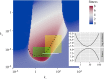

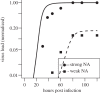
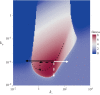
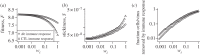
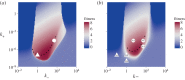
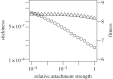


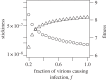
Similar articles
-
Extending the Stalk Enhances Immunogenicity of the Influenza Virus Neuraminidase.J Virol. 2019 Aug 28;93(18):e00840-19. doi: 10.1128/JVI.00840-19. Print 2019 Sep 15. J Virol. 2019. PMID: 31375573 Free PMC article.
-
Neuraminidase-Inhibiting Antibody Titers Correlate with Protection from Heterologous Influenza Virus Strains of the Same Neuraminidase Subtype.J Virol. 2018 Aug 16;92(17):e01006-18. doi: 10.1128/JVI.01006-18. Print 2018 Sep 1. J Virol. 2018. PMID: 29925654 Free PMC article.
-
Immune effects against influenza A virus and a novel DNA vaccine with co-expression of haemagglutinin- and neuraminidase-encoding genes.J Med Microbiol. 2009 Jul;58(Pt 7):845-854. doi: 10.1099/jmm.0.006825-0. Epub 2009 Jun 4. J Med Microbiol. 2009. PMID: 19498203
-
A new role of neuraminidase (NA) in the influenza virus life cycle: implication for developing NA inhibitors with novel mechanism of action.Rev Med Virol. 2016 Jul;26(4):242-50. doi: 10.1002/rmv.1879. Epub 2016 Apr 8. Rev Med Virol. 2016. PMID: 27061123 Free PMC article. Review.
-
Functional balance between haemagglutinin and neuraminidase in influenza virus infections.Rev Med Virol. 2002 May-Jun;12(3):159-66. doi: 10.1002/rmv.352. Rev Med Virol. 2002. PMID: 11987141 Review.
Cited by
-
Using thermodynamic parameters to calibrate a mechanistic dose-response for infection of a host by a virus.Microb Risk Anal. 2018 Apr;8:1-13. doi: 10.1016/j.mran.2018.01.002. Epub 2018 Jan 4. Microb Risk Anal. 2018. PMID: 32289059 Free PMC article.
-
Host heterogeneity affects both parasite transmission to and fitness on subsequent hosts.Philos Trans R Soc Lond B Biol Sci. 2017 May 5;372(1719):20160093. doi: 10.1098/rstb.2016.0093. Philos Trans R Soc Lond B Biol Sci. 2017. PMID: 28289260 Free PMC article.
-
A Sensitive Method for Detecting Zika Virus Antigen in Patients' Whole-Blood Specimens as an Alternative Diagnostic Approach.J Infect Dis. 2017 Jul 15;216(2):182-190. doi: 10.1093/infdis/jix276. J Infect Dis. 2017. PMID: 28586426 Free PMC article.
-
Towards a thermodynamic mechanistic model for the effect of temperature on arthropod vector competence for transmission of arboviruses.Microb Risk Anal. 2019 Aug;12:27-43. doi: 10.1016/j.mran.2019.03.001. Epub 2019 Mar 5. Microb Risk Anal. 2019. PMID: 32289057 Free PMC article.
-
Swine H1N1 Influenza Virus Variants with Enhanced Polymerase Activity and HA Stability Promote Airborne Transmission in Ferrets.J Virol. 2022 Apr 13;96(7):e0010022. doi: 10.1128/jvi.00100-22. Epub 2022 Mar 7. J Virol. 2022. PMID: 35254104 Free PMC article.
References
Publication types
MeSH terms
Substances
Grants and funding
LinkOut - more resources
Full Text Sources
Other Literature Sources
Medical