Mutations in the S6 gate isolate a late step in the activation pathway and reduce 4-AP sensitivity in shaker K(v) channel
- PMID: 24411245
- PMCID: PMC3907211
- DOI: 10.1016/j.bpj.2013.11.025
Mutations in the S6 gate isolate a late step in the activation pathway and reduce 4-AP sensitivity in shaker K(v) channel
Abstract
Kv channels detect changes in the membrane potential via their voltage-sensing domains (VSDs) that control the status of the S6 bundle crossing (BC) gate. The movement of the VSDs results in a transfer of the S4 gating charges across the cell membrane but only the last 10-20% of the total gating charge movement is associated with BC gate opening, which involves cooperative transition(s) in the subunits. Substituting the proline residue P475 in the S6 of the Shaker channel by a glycine or alanine causes a considerable shift in the voltage-dependence of the cooperative transition(s) of BC gate opening, effectively isolating the late gating charge component from the other gating charge that originates from earlier VSD movements. Interestingly, both mutations also abolished Shaker's sensitivity to 4-aminopyridine, which is a pharmacological tool to isolate the late gating charge component. The alanine substitution (that would promote a α-helical configuration compared to proline) resulted in the largest separation of both gating charge components; therefore, BC gate flexibility appears to be important for enabling the late cooperative step of channel opening.
Copyright © 2014 Biophysical Society. Published by Elsevier Inc. All rights reserved.
Figures
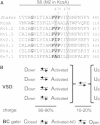
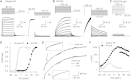
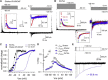
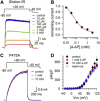
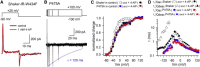
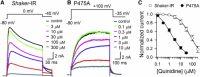
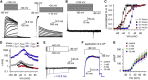
Similar articles
-
Alkanols inhibit voltage-gated K(+) channels via a distinct gating modifying mechanism that prevents gate opening.Sci Rep. 2015 Nov 30;5:17402. doi: 10.1038/srep17402. Sci Rep. 2015. PMID: 26616025 Free PMC article.
-
Status of the intracellular gate in the activated-not-open state of shaker K+ channels.J Gen Physiol. 2005 Nov;126(5):419-28. doi: 10.1085/jgp.200509385. J Gen Physiol. 2005. PMID: 16260836 Free PMC article.
-
KCNQ1 channels do not undergo concerted but sequential gating transitions in both the absence and the presence of KCNE1 protein.J Biol Chem. 2012 Oct 5;287(41):34212-24. doi: 10.1074/jbc.M112.364901. Epub 2012 Aug 20. J Biol Chem. 2012. PMID: 22908235 Free PMC article.
-
Involvement of the S4-S5 linker and the C-linker domain regions to voltage-gating in plant Shaker channels: comparison with animal HCN and Kv channels.Plant Signal Behav. 2014;9(10):e972892. doi: 10.4161/15592316.2014.972892. Plant Signal Behav. 2014. PMID: 25482770 Free PMC article. Review.
-
The EAG Voltage-Dependent K+ Channel Subfamily: Similarities and Differences in Structural Organization and Gating.Front Pharmacol. 2020 Apr 15;11:411. doi: 10.3389/fphar.2020.00411. eCollection 2020. Front Pharmacol. 2020. PMID: 32351384 Free PMC article. Review.
Cited by
-
Gambierol and n-alkanols inhibit Shaker Kv channel via distinct binding sites outside the K(+) pore.Toxicon. 2016 Sep 15;120:57-60. doi: 10.1016/j.toxicon.2016.07.017. Epub 2016 Jul 28. Toxicon. 2016. PMID: 27475861 Free PMC article.
-
Molecular rearrangements in S6 during slow inactivation in Shaker-IR potassium channels.J Gen Physiol. 2023 Jul 3;155(7):e202313352. doi: 10.1085/jgp.202313352. Epub 2023 May 22. J Gen Physiol. 2023. PMID: 37212728 Free PMC article.
-
Shaker-IR K+ channel gating in heavy water: Role of structural water molecules in inactivation.J Gen Physiol. 2021 Jun 7;153(6):e202012742. doi: 10.1085/jgp.202012742. Epub 2021 May 20. J Gen Physiol. 2021. PMID: 34014250 Free PMC article.
-
Inactivation of the Kv2.1 channel through electromechanical coupling.Nature. 2023 Oct;622(7982):410-417. doi: 10.1038/s41586-023-06582-8. Epub 2023 Sep 27. Nature. 2023. PMID: 37758949 Free PMC article.
-
Modulation of Closed-State Inactivation in Kv2.1/Kv6.4 Heterotetramers as Mechanism for 4-AP Induced Potentiation.PLoS One. 2015 Oct 27;10(10):e0141349. doi: 10.1371/journal.pone.0141349. eCollection 2015. PLoS One. 2015. PMID: 26505474 Free PMC article.
References
Publication types
MeSH terms
Substances
LinkOut - more resources
Full Text Sources
Other Literature Sources