Obesity and lipid stress inhibit carnitine acetyltransferase activity
- PMID: 24395925
- PMCID: PMC3966698
- DOI: 10.1194/jlr.M043448
Obesity and lipid stress inhibit carnitine acetyltransferase activity
Abstract
Carnitine acetyltransferase (CrAT) is a mitochondrial matrix enzyme that catalyzes the interconversion of acetyl-CoA and acetylcarnitine. Emerging evidence suggests that this enzyme functions as a positive regulator of total body glucose tolerance and muscle activity of pyruvate dehydrogenase (PDH), a mitochondrial enzyme complex that promotes glucose oxidation and is feedback inhibited by acetyl-CoA. Here, we used tandem mass spectrometry-based metabolic profiling to identify a negative relationship between CrAT activity and muscle content of lipid intermediates. CrAT specific activity was diminished in muscles from obese and diabetic rodents despite increased protein abundance. This reduction in enzyme activity was accompanied by muscle accumulation of long-chain acylcarnitines (LCACs) and acyl-CoAs and a decline in the acetylcarnitine/acetyl-CoA ratio. In vitro assays demonstrated that palmitoyl-CoA acts as a direct mixed-model inhibitor of CrAT. Similarly, in primary human myocytes grown in culture, nutritional and genetic manipulations that promoted mitochondrial influx of fatty acids resulted in accumulation of LCACs but a pronounced decrease of CrAT-derived short-chain acylcarnitines. These results suggest that lipid-induced antagonism of CrAT might contribute to decreased PDH activity and glucose disposal in the context of obesity and diabetes.
Keywords: acetyl-coenzyme A; acylcarnitines; diabetes; lipid metabolism; mitochondria; muscle.
Figures
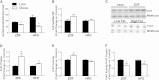
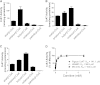
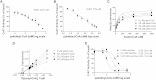
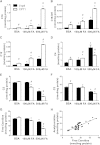
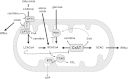
Similar articles
-
Muscle-specific deletion of carnitine acetyltransferase compromises glucose tolerance and metabolic flexibility.Cell Metab. 2012 May 2;15(5):764-77. doi: 10.1016/j.cmet.2012.04.005. Cell Metab. 2012. PMID: 22560225 Free PMC article.
-
Carnitine insufficiency caused by aging and overnutrition compromises mitochondrial performance and metabolic control.J Biol Chem. 2009 Aug 21;284(34):22840-52. doi: 10.1074/jbc.M109.032888. Epub 2009 Jun 24. J Biol Chem. 2009. PMID: 19553674 Free PMC article.
-
Substrate specificity of human carnitine acetyltransferase: Implications for fatty acid and branched-chain amino acid metabolism.Biochim Biophys Acta. 2013 Jun;1832(6):773-9. doi: 10.1016/j.bbadis.2013.02.012. Epub 2013 Feb 24. Biochim Biophys Acta. 2013. PMID: 23485643
-
Regulation by carnitine of myocardial fatty acid and carbohydrate metabolism under normal and pathological conditions.Basic Res Cardiol. 2000 Apr;95(2):75-83. doi: 10.1007/s003950050167. Basic Res Cardiol. 2000. PMID: 10826498 Review.
-
Role of CoA and acetyl-CoA in regulating cardiac fatty acid and glucose oxidation.Biochem Soc Trans. 2014 Aug;42(4):1043-51. doi: 10.1042/BST20140094. Biochem Soc Trans. 2014. PMID: 25110000 Review.
Cited by
-
Mass Spectrometry-Based Multiomics Identifies Metabolic Signatures of Sarcopenia in Rhesus Monkey Skeletal Muscle.J Proteome Res. 2024 Aug 2;23(8):2845-2856. doi: 10.1021/acs.jproteome.3c00474. Epub 2023 Nov 22. J Proteome Res. 2024. PMID: 37991985 Free PMC article.
-
A genetic screen identifies Crat as a regulator of pancreatic beta-cell insulin secretion.Mol Metab. 2020 Jul;37:100993. doi: 10.1016/j.molmet.2020.100993. Epub 2020 Apr 13. Mol Metab. 2020. PMID: 32298772 Free PMC article.
-
A Metabolomic Analysis of the Sex-Dependent Hispanic Paradox.Metabolites. 2021 Aug 20;11(8):552. doi: 10.3390/metabo11080552. Metabolites. 2021. PMID: 34436492 Free PMC article.
-
Metabolic changes induced by oral glucose tests in horses and their diagnostic use.J Vet Intern Med. 2021 Jan;35(1):597-605. doi: 10.1111/jvim.15992. Epub 2020 Dec 5. J Vet Intern Med. 2021. PMID: 33277752 Free PMC article.
-
Evidence of a preferred kinetic pathway in the carnitine acetyltransferase reaction.Arch Biochem Biophys. 2020 Sep 30;691:108507. doi: 10.1016/j.abb.2020.108507. Epub 2020 Jul 22. Arch Biochem Biophys. 2020. PMID: 32710884 Free PMC article.
References
-
- Muoio D. M., Noland R. C., Kovalik J. P., Seiler S. E., Davies M. N., DeBalsi K. L., Ilkayeva O. R., Stevens R. D., Kheterpal I., Zhang J., et al. 2012. Muscle-specific deletion of carnitine acetyltransferase compromises glucose tolerance and metabolic flexibility. Cell Metab. 15: 764–777. - PMC - PubMed
-
- Kerner J., Turkaly P. J., Minkler P. E., Hoppel C. L. 2001. Aging skeletal muscle mitochondria in the rat: decreased uncoupling protein-3 content. Am. J. Physiol. Endocrinol. Metab. 281: E1054–E1062. - PubMed
-
- An J., Muoio D. M., Shiota M., Fujimoto Y., Cline G. W., Shulman G. I., Koves T. R., Stevens R., Millington D., Newgard C. B. 2004. Hepatic expression of malonyl-CoA decarboxylase reverses muscle, liver and whole-animal insulin resistance. Nat. Med. 10: 268–274. - PubMed
-
- Haqq A. M., Lien L. F., Boan J., Arlotto M., Slentz C. A., Muehlbauer M. J., Rochon J., Gallup D., McMahon R. L., Bain J. R., et al. 2005. The Study of the Effects of Diet on Metabolism and Nutrition (STEDMAN) weight loss project: rationale and design. Contemp. Clin. Trials. 26: 616–625. - PubMed
Publication types
MeSH terms
Substances
Grants and funding
LinkOut - more resources
Full Text Sources
Other Literature Sources
Medical
Molecular Biology Databases
Miscellaneous