Novel septin 9 repeat motifs altered in neuralgic amyotrophy bind and bundle microtubules
- PMID: 24344182
- PMCID: PMC3871440
- DOI: 10.1083/jcb.201308068
Novel septin 9 repeat motifs altered in neuralgic amyotrophy bind and bundle microtubules
Abstract
Septin 9 (SEPT9) interacts with microtubules (MTs) and is mutated in hereditary neuralgic amyotrophy (HNA), an autosomal-dominant neuropathy. The mechanism of SEPT9 interaction with MTs and the molecular basis of HNA are unknown. Here, we show that the N-terminal domain of SEPT9 contains the novel repeat motifs K/R-x-x-E/D and R/K-R-x-E, which bind and bundle MTs by interacting with the acidic C-terminal tails of β-tubulin. Alanine scanning mutagenesis revealed that the K/R-R/x-x-E/D motifs pair electrostatically with one another and the tails of β-tubulin, enabling septin–septin interactions that link MTs together. SEPT9 isoforms lacking repeat motifs or containing the HNA-linked mutation R88W, which maps to the R/K-R-x-E motif, diminished intracellular MT bundling and impaired asymmetric neurite growth in PC-12 cells. Thus, the SEPT9 repeat motifs bind and bundle MTs, and thereby promote asymmetric neurite growth. These results provide the first insight into the mechanism of septin interaction with MTs and the molecular and cellular basis of HNA.
Figures
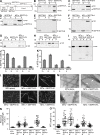
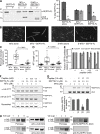
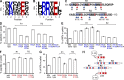
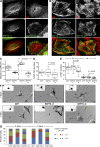
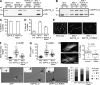
Similar articles
-
Mutations in SEPT9 cause hereditary neuralgic amyotrophy.Nat Genet. 2005 Oct;37(10):1044-6. doi: 10.1038/ng1649. Epub 2005 Sep 25. Nat Genet. 2005. PMID: 16186812
-
Phenotypic spectrum of hereditary neuralgic amyotrophy caused by the SEPT9 R88W mutation.J Neurol Neurosurg Psychiatry. 2010 Jan;81(1):94-6. doi: 10.1136/jnnp.2008.168260. J Neurol Neurosurg Psychiatry. 2010. PMID: 20019224
-
SEPT9 gene sequencing analysis reveals recurrent mutations in hereditary neuralgic amyotrophy.Neurology. 2009 May 19;72(20):1755-9. doi: 10.1212/WNL.0b013e3181a609e3. Neurology. 2009. PMID: 19451530 Free PMC article.
-
Inherited focal, episodic neuropathies: hereditary neuropathy with liability to pressure palsies and hereditary neuralgic amyotrophy.Neuromolecular Med. 2006;8(1-2):159-74. doi: 10.1385/NMM:8:1:159. Neuromolecular Med. 2006. PMID: 16775374 Review.
-
Conquering the complex world of human septins: implications for health and disease.Clin Genet. 2010 Jun;77(6):511-24. doi: 10.1111/j.1399-0004.2010.01392.x. Epub 2010 Feb 11. Clin Genet. 2010. PMID: 20236126 Free PMC article. Review.
Cited by
-
Septins promote stress fiber-mediated maturation of focal adhesions and renal epithelial motility.J Cell Biol. 2014 Oct 27;207(2):225-35. doi: 10.1083/jcb.201405050. J Cell Biol. 2014. PMID: 25349260 Free PMC article.
-
MLT1 links cytoskeletal asymmetry to organelle placement in chlamydomonas.Cytoskeleton (Hoboken). 2015 Mar;72(3):113-23. doi: 10.1002/cm.21220. Epub 2015 Apr 21. Cytoskeleton (Hoboken). 2015. PMID: 25809438 Free PMC article.
-
Septin 2/6/7 complexes tune microtubule plus-end growth and EB1 binding in a concentration- and filament-dependent manner.Mol Biol Cell. 2019 Nov 1;30(23):2913-2928. doi: 10.1091/mbc.E19-07-0362. Epub 2019 Oct 2. Mol Biol Cell. 2019. PMID: 31577529 Free PMC article.
-
Septin Remodeling During Mammalian Cytokinesis.Front Cell Dev Biol. 2021 Nov 4;9:768309. doi: 10.3389/fcell.2021.768309. eCollection 2021. Front Cell Dev Biol. 2021. PMID: 34805175 Free PMC article. Review.
-
Septin cooperation with tubulin polyglutamylation contributes to cancer cell adaptation to taxanes.Oncotarget. 2015 Nov 3;6(34):36063-80. doi: 10.18632/oncotarget.5373. Oncotarget. 2015. PMID: 26460824 Free PMC article.
References
-
- Bertin A., McMurray M.A., Grob P., Park S.S., Garcia G., III, Patanwala I., Ng H.L., Alber T., Thorner J., Nogales E.. 2008. Saccharomyces cerevisiae septins: supramolecular organization of heterooligomers and the mechanism of filament assembly. Proc. Natl. Acad. Sci. USA. 105:8274–8279. 10.1073/pnas.0803330105 - DOI - PMC - PubMed
Publication types
MeSH terms
Substances
Grants and funding
LinkOut - more resources
Full Text Sources
Other Literature Sources
Molecular Biology Databases
Research Materials