DNA sequence-dependent mechanics and protein-assisted bending in repressor-mediated loop formation
- PMID: 24231252
- PMCID: PMC3915735
- DOI: 10.1088/1478-3975/10/6/066005
DNA sequence-dependent mechanics and protein-assisted bending in repressor-mediated loop formation
Abstract
As the chief informational molecule of life, DNA is subject to extensive physical manipulations. The energy required to deform double-helical DNA depends on sequence, and this mechanical code of DNA influences gene regulation, such as through nucleosome positioning. Here we examine the sequence-dependent flexibility of DNA in bacterial transcription factor-mediated looping, a context for which the role of sequence remains poorly understood. Using a suite of synthetic constructs repressed by the Lac repressor and two well-known sequences that show large flexibility differences in vitro, we make precise statistical mechanical predictions as to how DNA sequence influences loop formation and test these predictions using in vivo transcription and in vitro single-molecule assays. Surprisingly, sequence-dependent flexibility does not affect in vivo gene regulation. By theoretically and experimentally quantifying the relative contributions of sequence and the DNA-bending protein HU to DNA mechanical properties, we reveal that bending by HU dominates DNA mechanics and masks intrinsic sequence-dependent flexibility. Such a quantitative understanding of how mechanical regulatory information is encoded in the genome will be a key step towards a predictive understanding of gene regulation at single-base pair resolution.
Figures
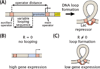
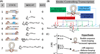
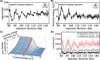
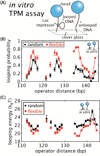
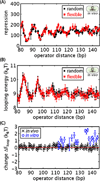
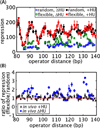
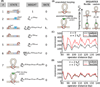
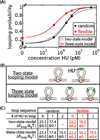
Similar articles
-
Analysis of in-vivo LacR-mediated gene repression based on the mechanics of DNA looping.PLoS One. 2006 Dec 27;1(1):e136. doi: 10.1371/journal.pone.0000136. PLoS One. 2006. PMID: 17205140 Free PMC article.
-
Engineered transcription activator-like effector dimer proteins confer DNA loop-dependent gene repression comparable to Lac repressor.Nucleic Acids Res. 2024 Sep 9;52(16):9996-10004. doi: 10.1093/nar/gkae656. Nucleic Acids Res. 2024. PMID: 39077947 Free PMC article.
-
Gene repression by minimal lac loops in vivo.Nucleic Acids Res. 2010 Dec;38(22):8072-82. doi: 10.1093/nar/gkq755. Nucleic Acids Res. 2010. PMID: 21149272 Free PMC article.
-
DNA looping in prokaryotes: experimental and theoretical approaches.J Bacteriol. 2013 Mar;195(6):1109-19. doi: 10.1128/JB.02038-12. Epub 2013 Jan 4. J Bacteriol. 2013. PMID: 23292776 Free PMC article. Review.
-
Lactose repressor protein: functional properties and structure.Prog Nucleic Acid Res Mol Biol. 1998;58:127-64. doi: 10.1016/s0079-6603(08)60035-5. Prog Nucleic Acid Res Mol Biol. 1998. PMID: 9308365 Review.
Cited by
-
DNA topology confers sequence specificity to nonspecific architectural proteins.Proc Natl Acad Sci U S A. 2014 Nov 25;111(47):16742-7. doi: 10.1073/pnas.1405016111. Epub 2014 Nov 10. Proc Natl Acad Sci U S A. 2014. PMID: 25385626 Free PMC article.
-
Predicting the impact of promoter variability on regulatory outputs.Sci Rep. 2015 Dec 17;5:18238. doi: 10.1038/srep18238. Sci Rep. 2015. PMID: 26675057 Free PMC article.
-
Multiplexed characterization of rationally designed promoter architectures deconstructs combinatorial logic for IPTG-inducible systems.Nat Commun. 2021 Jan 12;12(1):325. doi: 10.1038/s41467-020-20094-3. Nat Commun. 2021. PMID: 33436562 Free PMC article.
-
What controls DNA looping?Int J Mol Sci. 2014 Aug 27;15(9):15090-108. doi: 10.3390/ijms150915090. Int J Mol Sci. 2014. PMID: 25167135 Free PMC article.
-
Mapping DNA sequence to transcription factor binding energy in vivo.PLoS Comput Biol. 2019 Feb 4;15(2):e1006226. doi: 10.1371/journal.pcbi.1006226. eCollection 2019 Feb. PLoS Comput Biol. 2019. PMID: 30716072 Free PMC article.
References
-
- Rippe K, von Hippel PH, Langowski J. Action at a distance: DNA-looping and initiation of transcription. Trends Biochem Sci. 1995;20:500–506. - PubMed
-
- Hudson JM, Fried MG. Co-operative interactions between the catabolite gene activator protein and the lac repressor at the lactose promoter. J Mol Biol. 1990;214:381–396. - PubMed
-
- Ptashne M, Gann A. Transcriptional activation by recruitment. Nature. 1997;386:569–577. - PubMed
-
- Becker NA, Kahn JD, Maher LJ. Bacterial repression loops require enhanced DNA flexibility. J Mol Biol. 2005;349:716–730. - PubMed
Publication types
MeSH terms
Substances
Grants and funding
LinkOut - more resources
Full Text Sources
Other Literature Sources
Research Materials