The midbody ring scaffolds the abscission machinery in the absence of midbody microtubules
- PMID: 24217623
- PMCID: PMC3824018
- DOI: 10.1083/jcb.201306036
The midbody ring scaffolds the abscission machinery in the absence of midbody microtubules
Abstract
Abscission completes cytokinesis to form the two daughter cells. Although abscission could be organized from the inside out by the microtubule-based midbody or from the outside in by the contractile ring-derived midbody ring, it is assumed that midbody microtubules scaffold the abscission machinery. In this paper, we assess the contribution of midbody microtubules versus the midbody ring in the Caenorhabditis elegans embryo. We show that abscission occurs in two stages. First, the cytoplasm in the daughter cells becomes isolated, coincident with formation of the intercellular bridge; proper progression through this stage required the septins (a midbody ring component) but not the membrane-remodeling endosomal sorting complex required for transport (ESCRT) machinery. Second, the midbody and midbody ring are released into a specific daughter cell during the subsequent cell division; this stage required the septins and the ESCRT machinery. Surprisingly, midbody microtubules were dispensable for both stages. These results delineate distinct steps during abscission and highlight the central role of the midbody ring, rather than midbody microtubules, in their execution.
Figures
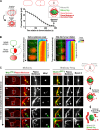
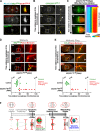
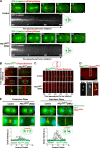
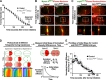
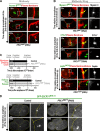
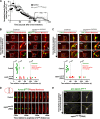
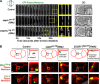
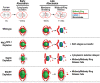
Similar articles
-
A Septin Double Ring Controls the Spatiotemporal Organization of the ESCRT Machinery in Cytokinetic Abscission.Curr Biol. 2019 Jul 8;29(13):2174-2182.e7. doi: 10.1016/j.cub.2019.05.050. Epub 2019 Jun 13. Curr Biol. 2019. PMID: 31204162 Free PMC article.
-
Membrane remodeling during embryonic abscission in Caenorhabditis elegans.J Cell Biol. 2017 May 1;216(5):1277-1286. doi: 10.1083/jcb.201607030. Epub 2017 Mar 21. J Cell Biol. 2017. PMID: 28325808 Free PMC article.
-
Actin, microtubule, septin and ESCRT filament remodeling during late steps of cytokinesis.Curr Opin Cell Biol. 2018 Feb;50:27-34. doi: 10.1016/j.ceb.2018.01.007. Epub 2018 Feb 10. Curr Opin Cell Biol. 2018. PMID: 29438904 Review.
-
Knowing when to cut and run: mechanisms that control cytokinetic abscission.Trends Cell Biol. 2013 Sep;23(9):433-41. doi: 10.1016/j.tcb.2013.04.006. Epub 2013 May 22. Trends Cell Biol. 2013. PMID: 23706391 Review.
-
Anillin-dependent organization of septin filaments promotes intercellular bridge elongation and Chmp4B targeting to the abscission site.Open Biol. 2014 Jan 22;4(1):130190. doi: 10.1098/rsob.130190. Open Biol. 2014. PMID: 24451548 Free PMC article.
Cited by
-
CYK-4 functions independently of its centralspindlin partner ZEN-4 to cellularize oocytes in germline syncytia.Elife. 2018 Jul 10;7:e36919. doi: 10.7554/eLife.36919. Elife. 2018. PMID: 29989548 Free PMC article.
-
A GAP that Divides.F1000Res. 2017 Oct 2;6:1788. doi: 10.12688/f1000research.12064.1. eCollection 2017. F1000Res. 2017. PMID: 29043078 Free PMC article. Review.
-
The cytokinetic midbody mediates asymmetric fate specification at mitotic exit during neural stem cell division.bioRxiv [Preprint]. 2024 Aug 28:2024.08.27.609974. doi: 10.1101/2024.08.27.609974. bioRxiv. 2024. PMID: 39253494 Free PMC article. Preprint.
-
Aurora B kinase promotes cytokinesis by inducing centralspindlin oligomers that associate with the plasma membrane.Dev Cell. 2015 Apr 20;33(2):204-15. doi: 10.1016/j.devcel.2015.03.015. Dev Cell. 2015. PMID: 25898168 Free PMC article.
-
Degron-tagged reporters probe membrane topology and enable the specific labelling of membrane-wrapped structures.Nat Commun. 2019 Aug 2;10(1):3490. doi: 10.1038/s41467-019-11442-z. Nat Commun. 2019. PMID: 31375709 Free PMC article.
References
Publication types
MeSH terms
Substances
Grants and funding
LinkOut - more resources
Full Text Sources
Other Literature Sources
Molecular Biology Databases