Bitter-sweet symphony: glycan-lectin interactions in virus biology
- PMID: 24188132
- PMCID: PMC7190080
- DOI: 10.1111/1574-6976.12052
Bitter-sweet symphony: glycan-lectin interactions in virus biology
Abstract
Glycans are carbohydrate modifications typically found on proteins or lipids, and can act as ligands for glycan-binding proteins called lectins. Glycans and lectins play crucial roles in the function of cells and organs, and in the immune system of animals and humans. Viral pathogens use glycans and lectins that are encoded by their own or the host genome for their replication and spread. Recent advances in glycobiological research indicate that glycans and lectins mediate key interactions at the virus-host interface, controlling viral spread and/or activation of the immune system. This review reflects on glycan-lectin interactions in the context of viral infection and antiviral immunity. A short introduction illustrates the nature of glycans and lectins, and conveys the basic principles of their interactions. Subsequently, examples are discussed highlighting specific glycan-lectin interactions and how they affect the progress of viral infections, either benefiting the host or the virus. Moreover, glycan and lectin variability and their potential biological consequences are discussed. Finally, the review outlines how recent advances in the glycan-lectin field might be transformed into promising new approaches to antiviral therapy.
Keywords: DC-SIGN; antiviral; collectin; galectin; hemagglutinin; receptor-destroying enzyme.
© 2013 Federation of European Microbiological Societies. Published by John Wiley & Sons Ltd. All rights reserved.
Figures
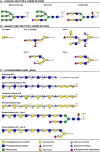
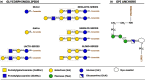
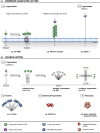
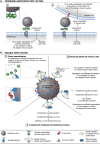
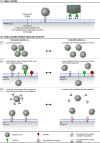
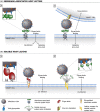
Similar articles
-
Glycan-Lectin Interactions in Cancer and Viral Infections and How to Disrupt Them.Int J Mol Sci. 2021 Sep 30;22(19):10577. doi: 10.3390/ijms221910577. Int J Mol Sci. 2021. PMID: 34638920 Free PMC article. Review.
-
Glycans from avian influenza virus are recognized by chicken dendritic cells and are targets for the humoral immune response in chicken.Mol Immunol. 2013 Dec;56(4):452-62. doi: 10.1016/j.molimm.2013.06.007. Epub 2013 Aug 1. Mol Immunol. 2013. PMID: 23911401
-
Legume Lectins with Different Specificities as Potential Glycan Probes for Pathogenic Enveloped Viruses.Cells. 2022 Jan 20;11(3):339. doi: 10.3390/cells11030339. Cells. 2022. PMID: 35159151 Free PMC article. Review.
-
Glycan-Gold Nanoparticles as Multifunctional Probes for Multivalent Lectin-Carbohydrate Binding: Implications for Blocking Virus Infection and Nanoparticle Assembly.J Am Chem Soc. 2020 Oct 21;142(42):18022-18034. doi: 10.1021/jacs.0c06793. Epub 2020 Sep 29. J Am Chem Soc. 2020. PMID: 32935985
-
SARS-CoV-2 Evolutionary Adaptation toward Host Entry and Recognition of Receptor O-Acetyl Sialylation in Virus-Host Interaction.Int J Mol Sci. 2020 Jun 26;21(12):4549. doi: 10.3390/ijms21124549. Int J Mol Sci. 2020. PMID: 32604730 Free PMC article. Review.
Cited by
-
Mammalian sugar-binding receptors: known functions and unexplored roles.FEBS J. 2019 May;286(10):1800-1814. doi: 10.1111/febs.14759. Epub 2019 Feb 6. FEBS J. 2019. PMID: 30657247 Free PMC article. Review.
-
Phenotypic and Functional Characterization of Monoclonal Antibodies with Specificity for Rhesus Macaque CD200, CD200R and Mincle.PLoS One. 2015 Oct 15;10(10):e0140689. doi: 10.1371/journal.pone.0140689. eCollection 2015. PLoS One. 2015. PMID: 26468886 Free PMC article.
-
An introduction to the sugar code.Histochem Cell Biol. 2017 Feb;147(2):111-117. doi: 10.1007/s00418-016-1521-9. Epub 2016 Dec 14. Histochem Cell Biol. 2017. PMID: 27975142
-
From examining the relationship between (corona)viral adhesins and galectins to glyco-perspectives.Biophys J. 2021 Mar 16;120(6):1031-1039. doi: 10.1016/j.bpj.2020.11.020. Epub 2020 Nov 26. Biophys J. 2021. PMID: 33248129 Free PMC article.
-
In Vitro Characterization of the Carbohydrate-Binding Agents HHA, GNA, and UDA as Inhibitors of Influenza A and B Virus Replication.Antimicrob Agents Chemother. 2021 Feb 17;65(3):e01732-20. doi: 10.1128/AAC.01732-20. Print 2021 Feb 17. Antimicrob Agents Chemother. 2021. PMID: 33288640 Free PMC article.
References
-
- Alymova IV, Taylor G, Portner A. (2005) Neuraminidase inhibitors as antiviral agents. Curr Drug Targets 5: 401–409. - PubMed
-
- Anders EM, Hartley CA, Reading PC, Ezekowitz RA. (1994) Complement-dependent neutralization of influenza virus by a serum mannose-binding lectin. J Gen Virol 75: 615–622. - PubMed
Publication types
MeSH terms
Substances
LinkOut - more resources
Full Text Sources
Other Literature Sources