Redox-dependent anti-inflammatory signaling actions of unsaturated fatty acids
- PMID: 24161076
- PMCID: PMC4030715
- DOI: 10.1146/annurev-physiol-021113-170341
Redox-dependent anti-inflammatory signaling actions of unsaturated fatty acids
Abstract
Unsaturated fatty acids are metabolized to reactive products that can act as pro- or anti-inflammatory signaling mediators. Electrophilic fatty acid species, including nitro- and oxo-containing fatty acids, display salutary anti-inflammatory and metabolic actions. Electrophilicity can be conferred by both enzymatic and oxidative reactions, via the homolytic addition of nitrogen dioxide to a double bond or via the formation of α,β-unsaturated carbonyl and epoxide substituents. The endogenous formation of electrophilic fatty acids is significant and influenced by diet, metabolic, and inflammatory reactions. Transcriptional regulatory proteins and enzymes can sense the redox status of the surrounding environment upon electrophilic fatty acid adduction of functionally significant, nucleophilic cysteines. Through this covalent and often reversible posttranslational modification, gene expression and metabolic responses are induced. At low concentrations, the pleiotropic signaling actions that are regulated by these protein targets suggest that some classes of electrophilic lipids may be useful for treating metabolic and inflammatory diseases.
Figures
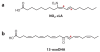
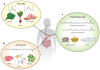
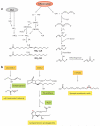
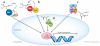
Similar articles
-
Primary saturation of α, β-unsaturated carbonyl containing fatty acids does not abolish electrophilicity.Chem Biol Interact. 2021 Dec 1;350:109689. doi: 10.1016/j.cbi.2021.109689. Epub 2021 Oct 8. Chem Biol Interact. 2021. PMID: 34634267 Free PMC article.
-
Nitro-fatty acids: formation, redox signaling, and therapeutic potential.Antioxid Redox Signal. 2013 Oct 10;19(11):1257-65. doi: 10.1089/ars.2012.5023. Epub 2012 Dec 20. Antioxid Redox Signal. 2013. PMID: 23256873 Review.
-
Olives and olive oil are sources of electrophilic fatty acid nitroalkenes.PLoS One. 2014 Jan 14;9(1):e84884. doi: 10.1371/journal.pone.0084884. eCollection 2014. PLoS One. 2014. PMID: 24454759 Free PMC article.
-
Generation and esterification of electrophilic fatty acid nitroalkenes in triacylglycerides.Free Radic Biol Med. 2015 Oct;87:113-24. doi: 10.1016/j.freeradbiomed.2015.05.033. Epub 2015 Jun 9. Free Radic Biol Med. 2015. PMID: 26066303 Free PMC article.
-
Anti-inflammatory signaling actions of electrophilic nitro-arachidonic acid in vascular cells and astrocytes.Arch Biochem Biophys. 2017 Mar 1;617:155-161. doi: 10.1016/j.abb.2016.10.003. Epub 2016 Oct 5. Arch Biochem Biophys. 2017. PMID: 27720684 Review.
Cited by
-
Lipoxin A4 yields an electrophilic 15-oxo metabolite that mediates FPR2 receptor-independent anti-inflammatory signaling.bioRxiv [Preprint]. 2024 Feb 7:2024.02.06.579101. doi: 10.1101/2024.02.06.579101. bioRxiv. 2024. PMID: 38370667 Free PMC article. Preprint.
-
Signature precursor and mature microRNAs in cervical ripening during gestational diabetes mellitus lead to pre-term labor and other impediments in future.J Diabetes Metab Disord. 2023 May 25;22(2):945-965. doi: 10.1007/s40200-023-01232-2. eCollection 2023 Dec. J Diabetes Metab Disord. 2023. PMID: 37975145 Free PMC article. Review.
-
Inflammation and Oxidative Stress Induced by Obesity, Gestational Diabetes, and Preeclampsia in Pregnancy: Role of High-Density Lipoproteins as Vectors for Bioactive Compounds.Antioxidants (Basel). 2023 Oct 23;12(10):1894. doi: 10.3390/antiox12101894. Antioxidants (Basel). 2023. PMID: 37891973 Free PMC article. Review.
-
Fatty Acids Composition and HIV Infection: Altered Levels of n-6 Polyunsaturated Fatty Acids Are Associated with Disease Progression.Viruses. 2023 Jul 23;15(7):1613. doi: 10.3390/v15071613. Viruses. 2023. PMID: 37515299 Free PMC article.
-
Meta-omics profiling of the gut-lung axis illuminates metabolic networks and host-microbial interactions associated with elevated lung elastance in a murine model of obese allergic asthma.Front Microbiomes. 2023;2:1153691. doi: 10.3389/frmbi.2023.1153691. Epub 2023 May 5. Front Microbiomes. 2023. PMID: 37293566 Free PMC article.
References
LITERATURE CITED
-
- Singh J, Petter RC, Baillie TA, Whitty A. The resurgence of covalent drugs. Nat. Rev. Drug Discov. 2011;10:307–17. - PubMed
-
- Bottcher C, Pollmann S. Plant oxylipins: plant responses to 12-oxo-phytodienoic acid are governed by its specific structural and functional properties. FEBS J. 2009;276:4693–704. - PubMed
-
- Gersch M, Kreuzer J, Sieber SA. Electrophilic natural products and their biological targets. Nat. Prod. Rep. 2012;29:659–82. - PubMed
-
- Singer P, Shapiro H, Theilla M, Anbar R, Singer J, Cohen J. Anti-inflammatory properties of omega-3 fatty acids in critical illness: novel mechanisms and an integrative perspective. Intensiv. Care Med. 2008;34:1580–92. - PubMed
References
Publication types
MeSH terms
Substances
Grants and funding
LinkOut - more resources
Full Text Sources
Other Literature Sources