Pore waters regulate ion permeation in a calcium release-activated calcium channel
- PMID: 24101457
- PMCID: PMC3808583
- DOI: 10.1073/pnas.1316969110
Pore waters regulate ion permeation in a calcium release-activated calcium channel
Abstract
The recent crystal structure of Orai, the pore unit of a calcium release-activated calcium (CRAC) channel, is used as the starting point for molecular dynamics and free-energy calculations designed to probe this channel's conduction properties. In free molecular dynamics simulations, cations localize preferentially at the extracellular channel entrance near the ring of Glu residues identified in the crystal structure, whereas anions localize in the basic intracellular half of the pore. To begin to understand ion permeation, the potential of mean force (PMF) was calculated for displacing a single Na(+) ion along the pore of the CRAC channel. The computed PMF indicates that the central hydrophobic region provides the major hindrance for ion diffusion along the permeation pathway, thereby illustrating the nonconducting nature of the crystal structure conformation. Strikingly, further PMF calculations demonstrate that the mutation V174A decreases the free energy barrier for conduction, rendering the channel effectively open. This seemingly dramatic effect of mutating a nonpolar residue for a smaller nonpolar residue in the pore hydrophobic region suggests an important role for the latter in conduction. Indeed, our computations show that even without significant channel-gating motions, a subtle change in the number of pore waters is sufficient to reshape the local electrostatic field and modulate the energetics of conduction, a result that rationalizes recent experimental findings. The present work suggests the activation mechanism for the wild-type CRAC channel is likely regulated by the number of pore waters and hence pore hydration governs the conductance.
Keywords: computer simulation; store-operated calcium entry.
Conflict of interest statement
The authors declare no conflict of interest.
Figures

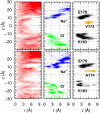
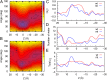
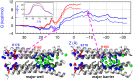
Similar articles
-
An energy-efficient gating mechanism in the acetylcholine receptor channel suggested by molecular and Brownian dynamics.Biophys J. 2006 Feb 1;90(3):799-810. doi: 10.1529/biophysj.105.067868. Epub 2005 Nov 11. Biophys J. 2006. PMID: 16284265 Free PMC article.
-
Orai1 mutations alter ion permeation and Ca2+-dependent fast inactivation of CRAC channels: evidence for coupling of permeation and gating.J Gen Physiol. 2007 Nov;130(5):525-40. doi: 10.1085/jgp.200709872. J Gen Physiol. 2007. PMID: 17968026 Free PMC article.
-
Counterion-assisted cation transport in a biological calcium channel.J Phys Chem B. 2014 Aug 14;118(32):9668-76. doi: 10.1021/jp5059897. Epub 2014 Aug 5. J Phys Chem B. 2014. PMID: 25068680
-
Permeation, selectivity and gating in store-operated CRAC channels.J Physiol. 2012 Sep 1;590(17):4179-91. doi: 10.1113/jphysiol.2012.233098. Epub 2012 May 14. J Physiol. 2012. PMID: 22586221 Free PMC article. Review.
-
The molecular physiology of CRAC channels.Immunol Rev. 2009 Sep;231(1):88-98. doi: 10.1111/j.1600-065X.2009.00820.x. Immunol Rev. 2009. PMID: 19754891 Free PMC article. Review.
Cited by
-
The basic residues in the Orai1 channel inner pore promote opening of the outer hydrophobic gate.J Gen Physiol. 2020 Jan 6;152(1):e201912397. doi: 10.1085/jgp.201912397. J Gen Physiol. 2020. PMID: 31816637 Free PMC article.
-
Hydrophobic gating in ion channels.J Mol Biol. 2015 Jan 16;427(1):121-30. doi: 10.1016/j.jmb.2014.07.030. Epub 2014 Aug 12. J Mol Biol. 2015. PMID: 25106689 Free PMC article. Review.
-
Blockage of Store-Operated Ca2+ Influx by Synta66 is Mediated by Direct Inhibition of the Ca2+ Selective Orai1 Pore.Cancers (Basel). 2020 Oct 6;12(10):2876. doi: 10.3390/cancers12102876. Cancers (Basel). 2020. PMID: 33036292 Free PMC article.
-
Mapping the functional anatomy of Orai1 transmembrane domains for CRAC channel gating.Proc Natl Acad Sci U S A. 2018 May 29;115(22):E5193-E5202. doi: 10.1073/pnas.1718373115. Epub 2018 May 14. Proc Natl Acad Sci U S A. 2018. PMID: 29760086 Free PMC article.
-
The open gate of the K(V)1.2 channel: quantum calculations show the key role of hydration.Biophys J. 2014 Feb 4;106(3):548-55. doi: 10.1016/j.bpj.2013.11.4495. Biophys J. 2014. PMID: 24507595 Free PMC article.
References
Publication types
MeSH terms
Substances
LinkOut - more resources
Full Text Sources
Other Literature Sources