Structure, dynamics and biophysics of the cytoplasmic protein-protein complexes of the bacterial phosphoenolpyruvate: sugar phosphotransferase system
- PMID: 24055245
- PMCID: PMC3831880
- DOI: 10.1016/j.tibs.2013.08.003
Structure, dynamics and biophysics of the cytoplasmic protein-protein complexes of the bacterial phosphoenolpyruvate: sugar phosphotransferase system
Abstract
The bacterial phosphotransferase system (PTS) couples phosphoryl transfer, via a series of bimolecular protein-protein interactions, to sugar transport across the membrane. The multitude of complexes in the PTS provides a paradigm for studying protein interactions, and for understanding how the same binding surface can specifically recognize a diverse array of targets. Fifteen years of work aimed at solving the solution structures of all soluble protein-protein complexes of the PTS has served as a test bed for developing NMR and integrated hybrid approaches to study larger complexes in solution and to probe transient, spectroscopically invisible states, including encounter complexes. We review these approaches, highlighting the problems that can be tackled with these methods, and summarize the current findings on protein interactions.
Keywords: NMR spectroscopy; bacterial phosphotransferase system; encounter complexes; hybrid methods in structure determination; protein–protein recognition; residual dipolar couplings; signal transduction; solution X-ray scattering; sparsely populated states.
Published by Elsevier Ltd.
Figures
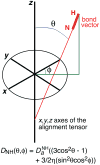
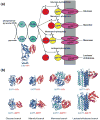
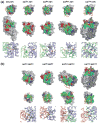
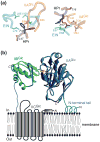
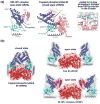
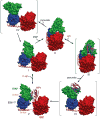
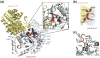
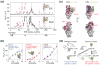
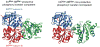
Similar articles
-
Three-dimensional structures of protein-protein complexes in the E. coli PTS.J Mol Microbiol Biotechnol. 2001 Jul;3(3):347-54. J Mol Microbiol Biotechnol. 2001. PMID: 11361064 Review.
-
Dynamic equilibrium between closed and partially closed states of the bacterial Enzyme I unveiled by solution NMR and X-ray scattering.Proc Natl Acad Sci U S A. 2015 Sep 15;112(37):11565-70. doi: 10.1073/pnas.1515366112. Epub 2015 Aug 24. Proc Natl Acad Sci U S A. 2015. PMID: 26305976 Free PMC article.
-
Carbohydrate Transport by Group Translocation: The Bacterial Phosphoenolpyruvate: Sugar Phosphotransferase System.Subcell Biochem. 2019;92:223-274. doi: 10.1007/978-3-030-18768-2_8. Subcell Biochem. 2019. PMID: 31214989 Review.
-
How phosphotransferase system-related protein phosphorylation regulates carbohydrate metabolism in bacteria.Microbiol Mol Biol Rev. 2006 Dec;70(4):939-1031. doi: 10.1128/MMBR.00024-06. Microbiol Mol Biol Rev. 2006. PMID: 17158705 Free PMC article. Review.
-
Solution structure of the phosphoryl transfer complex between the signal-transducing protein IIAGlucose and the cytoplasmic domain of the glucose transporter IICBGlucose of the Escherichia coli glucose phosphotransferase system.J Biol Chem. 2003 Jul 4;278(27):25191-206. doi: 10.1074/jbc.M302677200. Epub 2003 Apr 25. J Biol Chem. 2003. PMID: 12716891
Cited by
-
From Brown Seaweed to a Sustainable Microbial Feedstock for the Production of Riboflavin.Front Bioeng Biotechnol. 2022 Apr 12;10:863690. doi: 10.3389/fbioe.2022.863690. eCollection 2022. Front Bioeng Biotechnol. 2022. PMID: 35497351 Free PMC article.
-
Dibutyl phthalate alters the metabolic pathways of microbes in black soils.Sci Rep. 2018 Feb 8;8(1):2605. doi: 10.1038/s41598-018-21030-8. Sci Rep. 2018. PMID: 29422490 Free PMC article.
-
A Metabolic Widget Adjusts the Phosphoenolpyruvate-Dependent Fructose Influx in Pseudomonas putida.mSystems. 2016 Dec 6;1(6):e00154-16. doi: 10.1128/mSystems.00154-16. eCollection 2016 Nov-Dec. mSystems. 2016. PMID: 27933319 Free PMC article.
-
A Single Point Mutation Controls the Rate of Interconversion Between the g + and g - Rotamers of the Histidine 189 χ2 Angle That Activates Bacterial Enzyme I for Catalysis.Front Mol Biosci. 2021 Jul 8;8:699203. doi: 10.3389/fmolb.2021.699203. eCollection 2021. Front Mol Biosci. 2021. PMID: 34307459 Free PMC article.
-
Gut Microbiota Modulates Interactions Between Polychlorinated Biphenyls and Bile Acid Homeostasis.Toxicol Sci. 2018 Dec 1;166(2):269-287. doi: 10.1093/toxsci/kfy208. Toxicol Sci. 2018. PMID: 30496569 Free PMC article.
References
-
- Meadow ND, Fox DK, Roseman S. The bacterial phosphoenolpyruvate: glycose phosphotransferase system. Ann Rev Biochem. 1990;59:497–542. - PubMed
-
- Herzberg O, Klevit R. Unraveling a bacterial hexose transport pathway. Cuur Op Struct Biol. 1994;4:814–822. - PubMed
-
- Robillard GT, Broos J. Structure/function studies on the bacterial carbohydrate transporters, enzymes II, of the phosphoenolpyruvate-dependent phosphotransferase system. Biochim Biophys Acta. 1999;1422:73–104. - PubMed
Publication types
MeSH terms
Substances
Grants and funding
LinkOut - more resources
Full Text Sources
Other Literature Sources