Development and plasticity of the Drosophila larval neuromuscular junction
- PMID: 24014452
- PMCID: PMC3767937
- DOI: 10.1002/wdev.108
Development and plasticity of the Drosophila larval neuromuscular junction
Abstract
The Drosophila larval neuromuscular system is relatively simple, containing only 32 motor neurons in each abdominal hemisegment, and its neuromuscular junctions (NMJs) have been studied extensively. NMJ synapses exhibit developmental and functional plasticity while displaying stereotyped connectivity. Drosophila Type I NMJ synapses are glutamatergic, while the vertebrate NMJ uses acetylcholine as its primary neurotransmitter. The larval NMJ synapses use ionotropic glutamate receptors (GluRs) that are homologous to AMPA-type GluRs in the mammalian brain, and they have postsynaptic scaffolds that resemble those found in mammalian postsynaptic densities. These features make the Drosophila neuromuscular system an excellent genetic model for the study of excitatory synapses in the mammalian central nervous system. The first section of the review presents an overview of NMJ development. The second section describes genes that regulate NMJ development, including: (1) genes that positively and negatively regulate growth of the NMJ, (2) genes required for maintenance of NMJ bouton structure, (3) genes that modulate neuronal activity and alter NMJ growth, (4) genes involved in transsynaptic signaling at the NMJ. The third section describes genes that regulate acute plasticity, focusing on translational regulatory mechanisms. As this review is intended for a developmental biology audience, it does not cover NMJ electrophysiology in detail, and does not review genes for which mutations produce only electrophysiological but no structural phenotypes.
Copyright © 2013 Wiley Periodicals, Inc.
Figures
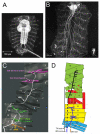
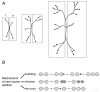
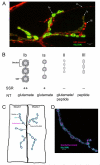
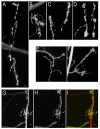
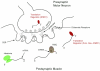
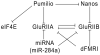
Similar articles
-
Identification of Mob2, a novel regulator of larval neuromuscular junction morphology, in natural populations of Drosophila melanogaster.Genetics. 2013 Nov;195(3):915-26. doi: 10.1534/genetics.113.156562. Epub 2013 Aug 26. Genetics. 2013. PMID: 23979583 Free PMC article.
-
Structural and Functional Synaptic Plasticity Induced by Convergent Synapse Loss in the Drosophila Neuromuscular Circuit.J Neurosci. 2021 Feb 17;41(7):1401-1417. doi: 10.1523/JNEUROSCI.1492-20.2020. Epub 2021 Jan 5. J Neurosci. 2021. PMID: 33402422 Free PMC article.
-
Maintenance of homeostatic plasticity at the Drosophila neuromuscular synapse requires continuous IP3-directed signaling.Elife. 2019 Jun 10;8:e39643. doi: 10.7554/eLife.39643. Elife. 2019. PMID: 31180325 Free PMC article.
-
Transmission, Development, and Plasticity of Synapses.Genetics. 2015 Oct;201(2):345-75. doi: 10.1534/genetics.115.176529. Genetics. 2015. PMID: 26447126 Free PMC article. Review.
-
Synaptic development: insights from Drosophila.Curr Opin Neurobiol. 2007 Feb;17(1):35-42. doi: 10.1016/j.conb.2007.01.001. Epub 2007 Jan 16. Curr Opin Neurobiol. 2007. PMID: 17229568 Review.
Cited by
-
Glia-derived exosomal miR-274 targets Sprouty in trachea and synaptic boutons to modulate growth and responses to hypoxia.Proc Natl Acad Sci U S A. 2019 Dec 3;116(49):24651-24661. doi: 10.1073/pnas.1902537116. Epub 2019 Oct 30. Proc Natl Acad Sci U S A. 2019. PMID: 31666321 Free PMC article.
-
Local BMP signaling: A sensor for synaptic activity that balances synapse growth and function.Curr Top Dev Biol. 2022;150:211-254. doi: 10.1016/bs.ctdb.2022.04.001. Epub 2022 May 18. Curr Top Dev Biol. 2022. PMID: 35817503 Free PMC article. Review.
-
Transsynaptic interactions between IgSF proteins DIP-α and Dpr10 are required for motor neuron targeting specificity.Elife. 2019 Feb 4;8:e42690. doi: 10.7554/eLife.42690. Elife. 2019. PMID: 30714906 Free PMC article.
-
Mucin-Type O-Glycosylation in the Drosophila Nervous System.Front Neuroanat. 2021 Oct 18;15:767126. doi: 10.3389/fnana.2021.767126. eCollection 2021. Front Neuroanat. 2021. PMID: 34733141 Free PMC article.
-
Transcriptional Reorganization of Drosophila Motor Neurons and Their Muscular Junctions toward a Neuroendocrine Phenotype by the bHLH Protein Dimmed.Front Mol Neurosci. 2017 Aug 14;10:260. doi: 10.3389/fnmol.2017.00260. eCollection 2017. Front Mol Neurosci. 2017. PMID: 28855860 Free PMC article.
References
-
- Tabuchi K, Sudhof TC. Structure and evolution of neurexin genes: insight into the mechanism of alternative splicing. Genomics. 2002 Jun;79(6):849–859. - PubMed
-
- Banovic D, Khorramshahi O, Owald D, et al. Drosophila neuroligin 1 promotes growth and postsynaptic differentiation at glutamatergic neuromuscular junctions. Neuron. 2010 Jun 10;66(5):724–738. - PubMed
Publication types
MeSH terms
Substances
Grants and funding
LinkOut - more resources
Full Text Sources
Other Literature Sources
Molecular Biology Databases
Research Materials