The responses of neural stem cells to the level of GSK-3 depend on the tissue of origin
- PMID: 23951407
- PMCID: PMC3744073
- DOI: 10.1242/bio.20131941
The responses of neural stem cells to the level of GSK-3 depend on the tissue of origin
Abstract
Neural stem cells (NSCs) can be obtained from a variety of sources, but not all NSCs exhibit the same characteristics. We have examined how the level of glycogen synthase kinase-3 activity regulates NSCs obtained from different sources: the mouse embryonic striatum, embryonic hippocampus, and mouse ES cells. Growth of striatal NSCs is enhanced by mild inhibition of GSK-3 but not by strong inhibition that is accompanied by Wnt/TCF transcriptional activation. In contrast, the growth of hippocampal NSCs is enhanced by both mild inhibition of GSK-3 as well as stronger inhibition. Active Wnt/TCF signaling, which occurs normally in the embryonic hippocampus, is required for growth of neural stem and progenitor cells. In the embryonic striatal germinal zone, however, TCF signaling is normally absent and its activation inhibits growth of NSCs from this region. Using a genetic model for progressive loss of GSK-3, we find that primitive ES cell-derived NSCs resemble striatal NSCs. That is, partial loss of GSK-3 alleles leads to an increase in NSCs while complete ablation of GSK-3, and activation of TCF-signaling, leads to their decline. Furthermore, expression of dominant negative TCF-4 in the GSK-3-null background was effective in blocking expression of Wnt-response genes and was also able to rescue neuronal gene expression. These results reveal that GSK-3 regulates NSCs by divergent pathways depending on the tissue of origin. The responses of these neural precursor cells may be contingent on baseline Wnt/TCF signaling occurring in a particular tissue.
Keywords: ES cells; Neural stem cells; Neurosphere assay; Wnt signaling.
Conflict of interest statement
Figures
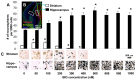
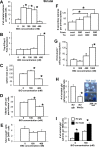
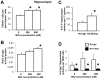
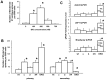
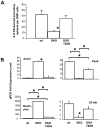
Similar articles
-
Glycogen Synthase Kinase-3β Regulates Equilibrium Between Neurogenesis and Gliogenesis in Rat Model of Parkinson's Disease: a Crosstalk with Wnt and Notch Signaling.Mol Neurobiol. 2018 Aug;55(8):6500-6517. doi: 10.1007/s12035-017-0860-4. Epub 2018 Jan 11. Mol Neurobiol. 2018. PMID: 29327199
-
BDNF promotes human neural stem cell growth via GSK-3β-mediated crosstalk with the wnt/β-catenin signaling pathway.Growth Factors. 2016 Feb;34(1-2):19-32. doi: 10.3109/08977194.2016.1157791. Epub 2016 May 4. Growth Factors. 2016. PMID: 27144323
-
Brain-derived Neurotrophic Factor Promotes Growth of Neurons and Neural Stem Cells Possibly by Triggering the Phosphoinositide 3-Kinase/ AKT/Glycogen Synthase Kinase-3β/β-catenin Pathway.CNS Neurol Disord Drug Targets. 2017;16(7):828-836. doi: 10.2174/1871527316666170518170422. CNS Neurol Disord Drug Targets. 2017. PMID: 28524001
-
New steps in the Wnt/beta-catenin signal transduction pathway.Recent Prog Horm Res. 2000;55:225-36. Recent Prog Horm Res. 2000. PMID: 11036939 Review.
-
Emerging Molecular Pathways Governing Dietary Regulation of Neural Stem Cells during Aging.Front Physiol. 2017 Jan 30;8:17. doi: 10.3389/fphys.2017.00017. eCollection 2017. Front Physiol. 2017. PMID: 28194114 Free PMC article. Review.
Cited by
-
Pediatric glioblastoma cells inhibit neurogenesis and promote astrogenesis, phenotypic transformation and migration of human neural progenitor cells within cocultures.Exp Cell Res. 2018 Jan 1;362(1):159-171. doi: 10.1016/j.yexcr.2017.11.013. Epub 2017 Nov 10. Exp Cell Res. 2018. PMID: 29129566 Free PMC article.
-
Overexpression of GSK-3β in Adult Tet-OFF GSK-3β Transgenic Mice, and Not During Embryonic or Postnatal Development, Induces Tau Phosphorylation, Neurodegeneration and Learning Deficits.Front Mol Neurosci. 2020 Sep 10;13:561470. doi: 10.3389/fnmol.2020.561470. eCollection 2020. Front Mol Neurosci. 2020. PMID: 33013321 Free PMC article.
-
Molecular Bases of Alzheimer's Disease and Neurodegeneration: The Role of Neuroglia.Aging Dis. 2018 Dec 4;9(6):1134-1152. doi: 10.14336/AD.2018.0201. eCollection 2018 Dec. Aging Dis. 2018. PMID: 30574424 Free PMC article. Review.
-
GSK3β Overexpression in Dentate Gyrus Neural Precursor Cells Expands the Progenitor Pool and Enhances Memory Skills.J Biol Chem. 2016 Apr 8;291(15):8199-213. doi: 10.1074/jbc.M115.674531. Epub 2016 Feb 17. J Biol Chem. 2016. PMID: 26887949 Free PMC article.
References
LinkOut - more resources
Full Text Sources
Other Literature Sources
Molecular Biology Databases