Widespread and enzyme-independent Nε-acetylation and Nε-succinylation of proteins in the chemical conditions of the mitochondrial matrix
- PMID: 23946487
- PMCID: PMC3790002
- DOI: 10.1074/jbc.M113.486753
Widespread and enzyme-independent Nε-acetylation and Nε-succinylation of proteins in the chemical conditions of the mitochondrial matrix
Abstract
Alterations in mitochondrial protein acetylation are implicated in the pathophysiology of diabetes, the metabolic syndrome, mitochondrial disorders, and cancer. However, a viable mechanism responsible for the widespread acetylation in mitochondria remains unknown. Here, we demonstrate that the physiologic pH and acyl-CoA concentrations of the mitochondrial matrix are sufficient to cause dose- and time-dependent, but enzyme-independent acetylation and succinylation of mitochondrial and nonmitochondrial proteins in vitro. These data suggest that protein acylation in mitochondria may be a chemical event facilitated by the alkaline pH and high concentrations of reactive acyl-CoAs present in the mitochondrial matrix. Although these results do not exclude the possibility of enzyme-mediated protein acylation in mitochondria, they demonstrate that such a mechanism may not be required in its unique chemical environment. These findings may have implications for the evolutionary roles that the mitochondria-localized SIRT3 deacetylase and SIRT5 desuccinylase have in the maintenance of metabolic health.
Keywords: Acetyl Coenzyme A; Acetylation; Metabolic Diseases; Metabolic Regulation; Mitochondrial Metabolism; Nonenzymatic; Sirtuins; Succinyl Coenzyme A; Succinylation; pH Regulation.
Figures
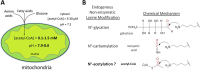
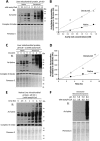
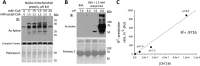
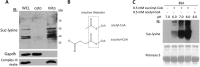
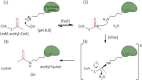
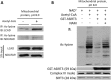
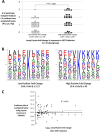
Similar articles
-
The ɛ-Amino Group of Protein Lysine Residues Is Highly Susceptible to Nonenzymatic Acylation by Several Physiological Acyl-CoA Thioesters.Chembiochem. 2015 Nov 2;16(16):2337-47. doi: 10.1002/cbic.201500364. Epub 2015 Sep 18. Chembiochem. 2015. PMID: 26382620
-
Mitochondrial protein acylation and intermediary metabolism: regulation by sirtuins and implications for metabolic disease.J Biol Chem. 2012 Dec 14;287(51):42436-43. doi: 10.1074/jbc.R112.404863. Epub 2012 Oct 18. J Biol Chem. 2012. PMID: 23086951 Free PMC article. Review.
-
The Mitochondrial Acylome Emerges: Proteomics, Regulation by Sirtuins, and Metabolic and Disease Implications.Cell Metab. 2018 Mar 6;27(3):497-512. doi: 10.1016/j.cmet.2018.01.016. Cell Metab. 2018. PMID: 29514063 Free PMC article. Review.
-
The Role of Mitochondrial Non-Enzymatic Protein Acylation in Ageing.PLoS One. 2016 Dec 29;11(12):e0168752. doi: 10.1371/journal.pone.0168752. eCollection 2016. PLoS One. 2016. PMID: 28033361 Free PMC article.
-
Substrates and regulation mechanisms for the human mitochondrial sirtuins Sirt3 and Sirt5.J Mol Biol. 2008 Oct 10;382(3):790-801. doi: 10.1016/j.jmb.2008.07.048. Epub 2008 Jul 25. J Mol Biol. 2008. PMID: 18680753
Cited by
-
Catalysis by protein acetyltransferase Gcn5.Biochim Biophys Acta Gene Regul Mech. 2021 Feb;1864(2):194627. doi: 10.1016/j.bbagrm.2020.194627. Epub 2020 Aug 22. Biochim Biophys Acta Gene Regul Mech. 2021. PMID: 32841743 Free PMC article. Review.
-
Sirtuin 2 Regulates Protein LactoylLys Modifications.Chembiochem. 2021 Jun 15;22(12):2102-2106. doi: 10.1002/cbic.202000883. Epub 2021 Apr 9. Chembiochem. 2021. PMID: 33725370 Free PMC article.
-
Site-specific reactivity of nonenzymatic lysine acetylation.ACS Chem Biol. 2015 Jan 16;10(1):122-8. doi: 10.1021/cb500848p. ACS Chem Biol. 2015. PMID: 25555129 Free PMC article.
-
Defining the orphan functions of lysine acetyltransferases.ACS Chem Biol. 2015 Jan 16;10(1):85-94. doi: 10.1021/cb500853p. ACS Chem Biol. 2015. PMID: 25591746 Free PMC article. Review.
-
Extreme Acetylation of the Cardiac Mitochondrial Proteome Does Not Promote Heart Failure.Circ Res. 2020 Sep 25;127(8):1094-1108. doi: 10.1161/CIRCRESAHA.120.317293. Epub 2020 Jul 14. Circ Res. 2020. PMID: 32660330 Free PMC article.
References
-
- Choudhary C., Kumar C., Gnad F., Nielsen M. L., Rehman M., Walther T. C., Olsen J. V., Mann M. (2009) Lysine acetylation targets protein complexes and co-regulates major cellular functions. Science 325, 834–840 - PubMed
-
- Strahl B. D., Allis C. D. (2000) The language of covalent histone modifications. Nature 403, 41–45 - PubMed
-
- Starai V. J., Celic I., Cole R. N., Boeke J. D., Escalante-Semerena J. C. (2002) Sir2-dependent activation of acetyl-CoA synthetase by deacetylation of active lysine. Science 298, 2390–2392 - PubMed
-
- Hebert A. S., Dittenhafer-Reed K. E., Yu W., Bailey D. J., Selen E. S., Boersma M. D., Carson J. J., Tonelli M., Balloon A. J., Higbee A. J., Westphall M. S., Pagliarini D. J., Prolla T. A., Assadi-Porter F., Roy S., Denu J. M., Coon J. J. (2013) Calorie restriction and SIRT3 trigger global reprogramming of the mitochondrial protein acetylome. Mol. Cell 49, 186–199 - PMC - PubMed
Publication types
MeSH terms
Substances
Grants and funding
LinkOut - more resources
Full Text Sources
Other Literature Sources