Twins, quadruplexes, and more: functional aspects of native and engineered RNA self-assembly in vivo
- PMID: 23914307
- PMCID: PMC3725660
- DOI: 10.1080/21553769.2012.761163
Twins, quadruplexes, and more: functional aspects of native and engineered RNA self-assembly in vivo
Abstract
The primacy and power of RNA in governing many processes of life has begun to be more fully appreciated in both the discovery and inventive sciences. A variety of RNA interactions regulate gene expression, and structural self-assembly underlies many of these processes. The understanding sparked by these discoveries has inspired and informed the engineering of novel RNA structures, control elements, and genetic circuits in cells. Many of these engineered systems are built up fundamentally from RNA-RNA interactions, often combining modular, rational design with functional selection and screening. It is therefore useful to review the particular class of RNA-based regulatory mechanisms that rely on RNA self-assembly either through homomeric (self-self) or heteromeric (self-nonself) RNA-RNA interactions. Structures and sequence elements within individual RNAs create a basis for the pairing interactions, and in some instances can even lead to the formation of RNA polymers. Example systems of dimers, multimers, and polymers are reviewed in this article in the context of natural systems, wherein the function and impact of self-assemblies are understood. Following this, a brief overview is presented of specific engineered RNA self-assembly systems implemented in vivo, with lessons learned from both discovery and engineering approaches to RNA-RNA self-assembly.
Keywords: RNA engineering; RNA structure; dimeric and oligomeric RNA; noncoding RNA; self-assembly.
Figures
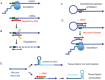
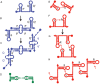
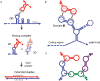
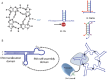
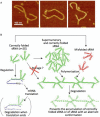
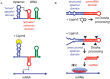
Similar articles
-
Macromolecular crowding: chemistry and physics meet biology (Ascona, Switzerland, 10-14 June 2012).Phys Biol. 2013 Aug;10(4):040301. doi: 10.1088/1478-3975/10/4/040301. Epub 2013 Aug 2. Phys Biol. 2013. PMID: 23912807
-
Engineering orthogonality in supramolecular polymers: from simple scaffolds to complex materials.Acc Chem Res. 2014 Aug 19;47(8):2405-16. doi: 10.1021/ar500128w. Epub 2014 Jun 6. Acc Chem Res. 2014. PMID: 24905869
-
Self-assembly of supramolecularly engineered polymers and their biomedical applications.Chem Commun (Camb). 2014 Oct 18;50(81):11994-2017. doi: 10.1039/c4cc03155e. Chem Commun (Camb). 2014. PMID: 25019489
-
Modular Tissue Assembly Strategies for Biofabrication of Engineered Cartilage.Ann Biomed Eng. 2017 Jan;45(1):100-114. doi: 10.1007/s10439-016-1609-3. Epub 2016 Apr 12. Ann Biomed Eng. 2017. PMID: 27073109 Review.
-
Self-organization in coordination-driven self-assembly.Acc Chem Res. 2009 Oct 20;42(10):1554-63. doi: 10.1021/ar900077c. Acc Chem Res. 2009. PMID: 19555073 Free PMC article. Review.
References
LinkOut - more resources
Full Text Sources