Multisite light-induced phosphorylation of the transcription factor PIF3 is necessary for both its rapid degradation and concomitant negative feedback modulation of photoreceptor phyB levels in Arabidopsis
- PMID: 23903316
- PMCID: PMC3753391
- DOI: 10.1105/tpc.113.112342
Multisite light-induced phosphorylation of the transcription factor PIF3 is necessary for both its rapid degradation and concomitant negative feedback modulation of photoreceptor phyB levels in Arabidopsis
Abstract
Plants constantly monitor informational light signals using sensory photoreceptors, which include the phytochrome (phy) family (phyA to phyE), and adjust their growth and development accordingly. Following light-induced nuclear translocation, photoactivated phy molecules bind to and induce rapid phosphorylation and degradation of phy-interacting basic Helix Loop Helix (bHLH) transcription factors (PIFs), such as PIF3, thereby regulating the expression of target genes. However, the mechanisms underlying the signal-relay process are still not fully understood. Here, using mass spectrometry, we identify multiple, in vivo, light-induced Ser/Thr phosphorylation sites in PIF3. Using transgenic expression of site-directed mutants of PIF3, we provide evidence that a set of these phosphorylation events acts collectively to trigger rapid degradation of the PIF3 protein in response to initial exposure of dark-grown seedlings to light. In addition, we show that phyB-induced PIF3 phosphorylation is also required for the known negative feedback modulation of phyB levels in prolonged light, potentially through codegradation of phyB and PIF3. This mutually regulatory intermolecular transaction thus provides a mechanism with the dual capacity to promote early, graded, or threshold regulation of the primary, PIF3-controlled transcriptional network in response to initial light exposure, and later, to attenuate global sensitivity to the light signal through reductions in photoreceptor levels upon prolonged exposure.
Figures
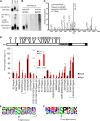
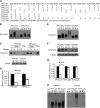
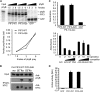
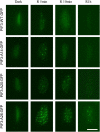
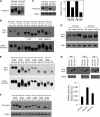
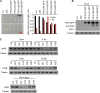
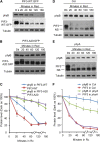
Similar articles
-
The Arabidopsis phytochrome-interacting factor PIF7, together with PIF3 and PIF4, regulates responses to prolonged red light by modulating phyB levels.Plant Cell. 2008 Feb;20(2):337-52. doi: 10.1105/tpc.107.052142. Epub 2008 Feb 5. Plant Cell. 2008. PMID: 18252845 Free PMC article.
-
Phytochrome signaling in green Arabidopsis seedlings: impact assessment of a mutually negative phyB-PIF feedback loop.Mol Plant. 2012 May;5(3):734-49. doi: 10.1093/mp/sss031. Epub 2012 Apr 5. Mol Plant. 2012. PMID: 22492120 Free PMC article.
-
Phytochrome induces rapid PIF5 phosphorylation and degradation in response to red-light activation.Plant Physiol. 2007 Nov;145(3):1043-51. doi: 10.1104/pp.107.105601. Epub 2007 Sep 7. Plant Physiol. 2007. PMID: 17827270 Free PMC article.
-
PIFs: systems integrators in plant development.Plant Cell. 2014 Jan;26(1):56-78. doi: 10.1105/tpc.113.120857. Epub 2014 Jan 30. Plant Cell. 2014. PMID: 24481072 Free PMC article. Review.
-
Multiple kinases promote light-induced degradation of PIF1.Plant Signal Behav. 2011 Aug;6(8):1119-21. doi: 10.4161/psb.6.8.16049. Epub 2011 Aug 1. Plant Signal Behav. 2011. PMID: 21758014 Free PMC article. Review.
Cited by
-
Phosphorylation of MdERF17 by MdMPK4 promotes apple fruit peel degreening during light/dark transitions.Plant Cell. 2022 Apr 26;34(5):1980-2000. doi: 10.1093/plcell/koac049. Plant Cell. 2022. PMID: 35166845 Free PMC article.
-
Phospho-mutant activity assays provide evidence for alternative phospho-regulation pathways of the transcription factor FER-LIKE IRON DEFICIENCY-INDUCED TRANSCRIPTION FACTOR.New Phytol. 2020 Jan;225(1):250-267. doi: 10.1111/nph.16168. Epub 2019 Oct 13. New Phytol. 2020. PMID: 31487399 Free PMC article.
-
PHYTOCHROME INTERACTING FACTOR8 Inhibits Phytochrome A-Mediated Far-Red Light Responses in Arabidopsis.Plant Cell. 2020 Jan;32(1):186-205. doi: 10.1105/tpc.19.00515. Epub 2019 Nov 15. Plant Cell. 2020. PMID: 31732705 Free PMC article.
-
Toward Multiplexed Optogenetic Circuits.Front Bioeng Biotechnol. 2022 Jan 5;9:804563. doi: 10.3389/fbioe.2021.804563. eCollection 2021. Front Bioeng Biotechnol. 2022. PMID: 35071213 Free PMC article. Review.
-
Identification of JAZ-interacting MYC transcription factors involved in latex drainage in Hevea brasiliensis.Sci Rep. 2018 Jan 17;8(1):909. doi: 10.1038/s41598-018-19206-3. Sci Rep. 2018. PMID: 29343866 Free PMC article.
References
-
- Al-Sady B., Ni W., Kircher S., Schäfer E., Quail P.H. (2006). Photoactivated phytochrome induces rapid PIF3 phosphorylation prior to proteasome-mediated degradation. Mol. Cell 23: 439–446 - PubMed
-
- Bauer D., Viczián A., Kircher S., Nobis T., Nitschke R., Kunkel T., Panigrahi K.C., Adám E., Fejes E., Schäfer E., Nagy F. (2004). Constitutive photomorphogenesis 1 and multiple photoreceptors control degradation of phytochrome interacting factor 3, a transcription factor required for light signaling in Arabidopsis. Plant Cell 16: 1433–1445 - PMC - PubMed
Publication types
MeSH terms
Substances
Grants and funding
LinkOut - more resources
Full Text Sources
Other Literature Sources
Molecular Biology Databases