Molecular paleontology and complexity in the last eukaryotic common ancestor
- PMID: 23895660
- PMCID: PMC3791482
- DOI: 10.3109/10409238.2013.821444
Molecular paleontology and complexity in the last eukaryotic common ancestor
Abstract
Eukaryogenesis, the origin of the eukaryotic cell, represents one of the fundamental evolutionary transitions in the history of life on earth. This event, which is estimated to have occurred over one billion years ago, remains rather poorly understood. While some well-validated examples of fossil microbial eukaryotes for this time frame have been described, these can provide only basic morphology and the molecular machinery present in these organisms has remained unknown. Complete and partial genomic information has begun to fill this gap, and is being used to trace proteins and cellular traits to their roots and to provide unprecedented levels of resolution of structures, metabolic pathways and capabilities of organisms at these earliest points within the eukaryotic lineage. This is essentially allowing a molecular paleontology. What has emerged from these studies is spectacular cellular complexity prior to expansion of the eukaryotic lineages. Multiple reconstructed cellular systems indicate a very sophisticated biology, which by implication arose following the initial eukaryogenesis event but prior to eukaryotic radiation and provides a challenge in terms of explaining how these early eukaryotes arose and in understanding how they lived. Here, we provide brief overviews of several cellular systems and the major emerging conclusions, together with predictions for subsequent directions in evolution leading to extant taxa. We also consider what these reconstructions suggest about the life styles and capabilities of these earliest eukaryotes and the period of evolution between the radiation of eukaryotes and the eukaryogenesis event itself.
Figures
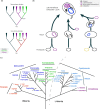
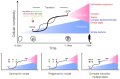
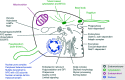
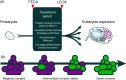
Similar articles
-
The changing view of eukaryogenesis - fossils, cells, lineages and how they all come together.J Cell Sci. 2016 Oct 15;129(20):3695-3703. doi: 10.1242/jcs.178566. Epub 2016 Sep 26. J Cell Sci. 2016. PMID: 27672020 Review.
-
The emerging view on the origin and early evolution of eukaryotic cells.Nature. 2024 Sep;633(8029):295-305. doi: 10.1038/s41586-024-07677-6. Epub 2024 Sep 11. Nature. 2024. PMID: 39261613 Review.
-
Origin and Early Evolution of the Eukaryotic Cell.Annu Rev Microbiol. 2021 Oct 8;75:631-647. doi: 10.1146/annurev-micro-090817-062213. Epub 2021 Aug 3. Annu Rev Microbiol. 2021. PMID: 34343017
-
Frameworks for Interpreting the Early Fossil Record of Eukaryotes.Annu Rev Microbiol. 2023 Sep 15;77:173-191. doi: 10.1146/annurev-micro-032421-113254. Annu Rev Microbiol. 2023. PMID: 37713454 Review.
-
Shedding light on the expansion and diversification of the Cdc48 protein family during the rise of the eukaryotic cell.BMC Evol Biol. 2016 Oct 18;16(1):215. doi: 10.1186/s12862-016-0790-1. BMC Evol Biol. 2016. PMID: 27756227 Free PMC article.
Cited by
-
Timing the origin of eukaryotic cellular complexity with ancient duplications.Nat Ecol Evol. 2021 Jan;5(1):92-100. doi: 10.1038/s41559-020-01320-z. Epub 2020 Oct 26. Nat Ecol Evol. 2021. PMID: 33106602 Free PMC article.
-
Apicomplexa Cell Cycles: Something Old, Borrowed, Lost, and New.Trends Parasitol. 2018 Sep;34(9):759-771. doi: 10.1016/j.pt.2018.07.006. Epub 2018 Aug 2. Trends Parasitol. 2018. PMID: 30078701 Free PMC article. Review.
-
Intronic and plasmid-derived regions contribute to the large mitochondrial genome sizes of Agaricomycetes.Curr Genet. 2014 Nov;60(4):303-13. doi: 10.1007/s00294-014-0436-z. Epub 2014 Jul 11. Curr Genet. 2014. PMID: 25011705 Free PMC article.
-
Lost world of complex life and the late rise of the eukaryotic crown.Nature. 2023 Jun;618(7966):767-773. doi: 10.1038/s41586-023-06170-w. Epub 2023 Jun 7. Nature. 2023. PMID: 37286610
-
Ancient eukaryotic protein interactions illuminate modern genetic traits and disorders.bioRxiv [Preprint]. 2024 May 29:2024.05.26.595818. doi: 10.1101/2024.05.26.595818. bioRxiv. 2024. PMID: 38853926 Free PMC article. Preprint.
References
-
- Alber F, Dokudovskaya S, Veenhoff LM, et al. The molecular architecture of the nuclear pore complex. Nature. 2007;450:695–701. - PubMed
-
- Alberts B, Johnson A, Lewis J, et al. Molecular biology of the cell. 4th. New York: Garland Science; 2002.
-
- Allen JW, Ferguson SJ, Ginger ML. Distinctive biochemistry in the trypanosome mitochondrial intermembrane space suggests a model for stepwise evolution of the MIA pathway for import of cysteine-rich proteins. FEBS Lett. 2008;582:2817–25. - PubMed
-
- Alsford S, Kawahara T, Isamah C, Horn D. A sirtuin in the African trypanosome is involved in both DNA repair and telomeric gene silencing but is not required for antigenic variation. Mol Microbiol. 2007;63:724–7. - PubMed
Publication types
MeSH terms
Grants and funding
LinkOut - more resources
Full Text Sources
Other Literature Sources
Miscellaneous