Protein S-mycothiolation functions as redox-switch and thiol protection mechanism in Corynebacterium glutamicum under hypochlorite stress
- PMID: 23886307
- PMCID: PMC3901351
- DOI: 10.1089/ars.2013.5423
Protein S-mycothiolation functions as redox-switch and thiol protection mechanism in Corynebacterium glutamicum under hypochlorite stress
Abstract
Aims: Protein S-bacillithiolation was recently discovered as important thiol protection and redox-switch mechanism in response to hypochlorite stress in Firmicutes bacteria. Here we used transcriptomics to analyze the NaOCl stress response in the mycothiol (MSH)-producing Corynebacterium glutamicum. We further applied thiol-redox proteomics and mass spectrometry (MS) to identify protein S-mycothiolation.
Results: Transcriptomics revealed the strong upregulation of the disulfide stress σ(H) regulon by NaOCl stress in C. glutamicum, including genes for the anti sigma factor (rshA), the thioredoxin and MSH pathways (trxB1, trxC, cg1375, trxB, mshC, mca, mtr) that maintain the redox balance. We identified 25 S-mycothiolated proteins in NaOCl-treated cells by liquid chromatography-tandem mass spectrometry (LC-MS/MS), including 16 proteins that are reversibly oxidized by NaOCl in the thiol-redox proteome. The S-mycothiolome includes the methionine synthase (MetE), the maltodextrin phosphorylase (MalP), the myoinositol-1-phosphate synthase (Ino1), enzymes for the biosynthesis of nucleotides (GuaB1, GuaB2, PurL, NadC), and thiamine (ThiD), translation proteins (TufA, PheT, RpsF, RplM, RpsM, RpsC), and antioxidant enzymes (Tpx, Gpx, MsrA). We further show that S-mycothiolation of the thiol peroxidase (Tpx) affects its peroxiredoxin activity in vitro that can be restored by mycoredoxin1. LC-MS/MS analysis further identified 8 proteins with S-cysteinylations in the mshC mutant suggesting that cysteine can be used for S-thiolations in the absence of MSH.
Innovation and conclusion: We identified widespread protein S-mycothiolations in the MSH-producing C. glutamicum and demonstrate that S-mycothiolation reversibly affects the peroxidase activity of Tpx. Interestingly, many targets are conserved S-thiolated across bacillithiol- and MSH-producing bacteria, which could become future drug targets in related pathogenic Gram-positives.
Figures
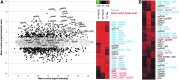
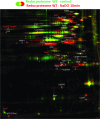
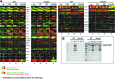
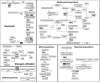
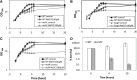
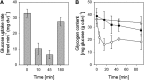
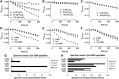
Similar articles
-
Monitoring global protein thiol-oxidation and protein S-mycothiolation in Mycobacterium smegmatis under hypochlorite stress.Sci Rep. 2017 Apr 26;7(1):1195. doi: 10.1038/s41598-017-01179-4. Sci Rep. 2017. PMID: 28446771 Free PMC article.
-
S-bacillithiolation protects against hypochlorite stress in Bacillus subtilis as revealed by transcriptomics and redox proteomics.Mol Cell Proteomics. 2011 Nov;10(11):M111.009506. doi: 10.1074/mcp.M111.009506. Epub 2011 Jul 11. Mol Cell Proteomics. 2011. PMID: 21749987 Free PMC article.
-
Redox regulation by reversible protein S-thiolation in Gram-positive bacteria.Redox Biol. 2019 Jan;20:130-145. doi: 10.1016/j.redox.2018.08.017. Epub 2018 Aug 24. Redox Biol. 2019. PMID: 30308476 Free PMC article. Review.
-
S-bacillithiolation protects conserved and essential proteins against hypochlorite stress in firmicutes bacteria.Antioxid Redox Signal. 2013 Apr 10;18(11):1273-95. doi: 10.1089/ars.2012.4686. Epub 2012 Oct 18. Antioxid Redox Signal. 2013. PMID: 22938038 Free PMC article.
-
Redox regulation by reversible protein S-thiolation in bacteria.Front Microbiol. 2015 Mar 16;6:187. doi: 10.3389/fmicb.2015.00187. eCollection 2015. Front Microbiol. 2015. PMID: 25852656 Free PMC article. Review.
Cited by
-
Overexpression of Mycothiol Disulfide Reductase Enhances Corynebacterium glutamicum Robustness by Modulating Cellular Redox Homeostasis and Antioxidant Proteins under Oxidative Stress.Sci Rep. 2016 Jul 7;6:29491. doi: 10.1038/srep29491. Sci Rep. 2016. PMID: 27383057 Free PMC article.
-
Methionine sulfoxide reductase B from Corynebacterium diphtheriae catalyzes sulfoxide reduction via an intramolecular disulfide cascade.J Biol Chem. 2020 Mar 13;295(11):3664-3677. doi: 10.1074/jbc.RA119.012438. Epub 2020 Jan 28. J Biol Chem. 2020. PMID: 31992594 Free PMC article.
-
Redox-Sensing Under Hypochlorite Stress and Infection Conditions by the Rrf2-Family Repressor HypR in Staphylococcus aureus.Antioxid Redox Signal. 2018 Sep 1;29(7):615-636. doi: 10.1089/ars.2017.7354. Epub 2018 Jan 30. Antioxid Redox Signal. 2018. PMID: 29237286 Free PMC article.
-
Corynebacterium glutamicum methionine sulfoxide reductase A uses both mycoredoxin and thioredoxin for regeneration and oxidative stress resistance.Appl Environ Microbiol. 2015 Apr;81(8):2781-96. doi: 10.1128/AEM.04221-14. Epub 2015 Feb 13. Appl Environ Microbiol. 2015. PMID: 25681179 Free PMC article.
-
Redox Regulation of the Quorum-sensing Transcription Factor AgrA by Coenzyme A.Antioxidants (Basel). 2021 May 25;10(6):841. doi: 10.3390/antiox10060841. Antioxidants (Basel). 2021. PMID: 34070323 Free PMC article.
References
Publication types
MeSH terms
Substances
Grants and funding
LinkOut - more resources
Full Text Sources
Other Literature Sources
Miscellaneous