Arabidopsis CURVATURE THYLAKOID1 proteins modify thylakoid architecture by inducing membrane curvature
- PMID: 23839788
- PMCID: PMC3753390
- DOI: 10.1105/tpc.113.113118
Arabidopsis CURVATURE THYLAKOID1 proteins modify thylakoid architecture by inducing membrane curvature
Abstract
Chloroplasts of land plants characteristically contain grana, cylindrical stacks of thylakoid membranes. A granum consists of a core of appressed membranes, two stroma-exposed end membranes, and margins, which connect pairs of grana membranes at their lumenal sides. Multiple forces contribute to grana stacking, but it is not known how the extreme curvature at margins is generated and maintained. We report the identification of the CURVATURE THYLAKOID1 (CURT1) protein family, conserved in plants and cyanobacteria. The four Arabidopsis thaliana CURT1 proteins (CURT1A, B, C, and D) oligomerize and are highly enriched at grana margins. Grana architecture is correlated with the CURT1 protein level, ranging from flat lobe-like thylakoids with considerably fewer grana margins in plants without CURT1 proteins to an increased number of membrane layers (and margins) in grana at the expense of grana diameter in overexpressors of CURT1A. The endogenous CURT1 protein in the cyanobacterium Synechocystis sp PCC6803 can be partially replaced by its Arabidopsis counterpart, indicating that the function of CURT1 proteins is evolutionary conserved. In vitro, Arabidopsis CURT1A proteins oligomerize and induce tubulation of liposomes, implying that CURT1 proteins suffice to induce membrane curvature. We therefore propose that CURT1 proteins modify thylakoid architecture by inducing membrane curvature at grana margins.
Figures
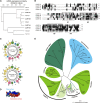
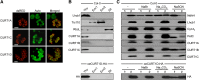
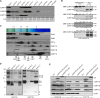
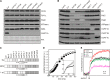
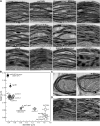
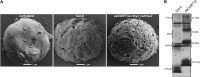
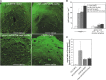
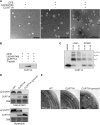
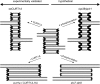
Similar articles
-
Fine-Tuning of Photosynthesis Requires CURVATURE THYLAKOID1-Mediated Thylakoid Plasticity.Plant Physiol. 2018 Mar;176(3):2351-2364. doi: 10.1104/pp.17.00863. Epub 2018 Jan 26. Plant Physiol. 2018. PMID: 29374108 Free PMC article.
-
Curvature thylakoid 1 proteins modulate prolamellar body morphology and promote organized thylakoid biogenesis in Arabidopsis thaliana.Proc Natl Acad Sci U S A. 2021 Oct 19;118(42):e2113934118. doi: 10.1073/pnas.2113934118. Proc Natl Acad Sci U S A. 2021. PMID: 34654749 Free PMC article.
-
Thylakoid-Bound Polysomes and a Dynamin-Related Protein, FZL, Mediate Critical Stages of the Linear Chloroplast Biogenesis Program in Greening Arabidopsis Cotyledons.Plant Cell. 2018 Jul;30(7):1476-1495. doi: 10.1105/tpc.17.00972. Epub 2018 Jun 7. Plant Cell. 2018. PMID: 29880711 Free PMC article.
-
CURT1,CAAD-containing aaRSs, thylakoid curvature and gene translation.Trends Plant Sci. 2014 Feb;19(2):63-6. doi: 10.1016/j.tplants.2013.12.004. Epub 2013 Dec 30. Trends Plant Sci. 2014. PMID: 24388270 Review.
-
Structure and dynamics of thylakoids in land plants.J Exp Bot. 2014 May;65(8):1955-72. doi: 10.1093/jxb/eru090. Epub 2014 Mar 12. J Exp Bot. 2014. PMID: 24622954 Review.
Cited by
-
Proteomic Insight into the Response of Arabidopsis Chloroplasts to Darkness.PLoS One. 2016 May 3;11(5):e0154235. doi: 10.1371/journal.pone.0154235. eCollection 2016. PLoS One. 2016. PMID: 27137770 Free PMC article.
-
Grana-Localized Proteins, RIQ1 and RIQ2, Affect the Organization of Light-Harvesting Complex II and Grana Stacking in Arabidopsis.Plant Cell. 2016 Sep;28(9):2261-2275. doi: 10.1105/tpc.16.00296. Epub 2016 Sep 6. Plant Cell. 2016. PMID: 27600538 Free PMC article.
-
Hydrocarbons Are Essential for Optimal Cell Size, Division, and Growth of Cyanobacteria.Plant Physiol. 2016 Nov;172(3):1928-1940. doi: 10.1104/pp.16.01205. Epub 2016 Oct 5. Plant Physiol. 2016. PMID: 27707888 Free PMC article.
-
Quality control of PSII: behavior of PSII in the highly crowded grana thylakoids under excessive light.Plant Cell Physiol. 2014 Jul;55(7):1206-15. doi: 10.1093/pcp/pcu043. Epub 2014 Mar 7. Plant Cell Physiol. 2014. PMID: 24610582 Free PMC article. Review.
-
Recent advances in understanding photosynthesis.F1000Res. 2016 Dec 21;5:2890. doi: 10.12688/f1000research.9744.1. eCollection 2016. F1000Res. 2016. PMID: 28105322 Free PMC article. Review.
References
-
- Albertsson P.A., Andreasson E. (2004). The constant proportion of grana and stroma lamellae in plant chloroplasts. Physiol. Plant. 121: 334–342 - PubMed
-
- Allen J.F., Forsberg J. (2001). Molecular recognition in thylakoid structure and function. Trends Plant Sci. 6: 317–326 - PubMed
-
- Anderson J.M. (1986). Photoregulation of the composition, function, and structure of thylakoid membranes. Annu. Rev. Plant Physiol. Plant Mol. Biol. 37: 93–136
-
- Anderson J.M., Chow W.S., De Las Rivas J. (2008). Dynamic flexibility in the structure and function of photosystem II in higher plant thylakoid membranes: The grana enigma. Photosynth. Res. 98: 575–587 - PubMed
Publication types
MeSH terms
Substances
LinkOut - more resources
Full Text Sources
Other Literature Sources
Molecular Biology Databases