Direct imaging reveals stable, micrometer-scale lipid domains that segregate proteins in live cells
- PMID: 23836928
- PMCID: PMC3704982
- DOI: 10.1083/jcb.201301039
Direct imaging reveals stable, micrometer-scale lipid domains that segregate proteins in live cells
Abstract
It has been proposed that membrane rafts, which are sterol- and sphingolipid-enriched liquid-ordered (Lo) domains, segregate proteins in membranes and play critical roles in numerous processes in cells. However, rafts remain controversial because they are difficult to observe in cells without invasive methods and seem to be very small (nanoscale) and short lived, leading many to question whether they exist or are physiologically relevant. In this paper, we show that micrometer-scale, stable lipid domains formed in the yeast vacuole membrane in response to nutrient deprivation, changes in the pH of the growth medium, and other stresses. All vacuolar membrane proteins tested segregated to one of two domains. These domains formed quasi-symmetrical patterns strikingly similar to those found in liposomes containing coexisting Lo and liquid-disordered regions. Indeed, we found that one of these domains is probably sterol enriched and Lo. Domain formation was shown to be regulated by the pH-responsive Rim101 signaling pathway and may also require vesicular trafficking to vacuoles.
Figures
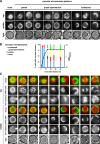
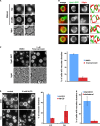
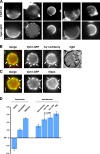
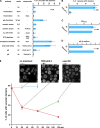
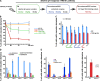
Similar articles
-
Lipids and lipid domains of the yeast vacuole.Biochem Soc Trans. 2018 Oct 19;46(5):1047-1054. doi: 10.1042/BST20180120. Epub 2018 Sep 20. Biochem Soc Trans. 2018. PMID: 30242116 Review.
-
Roles for L o microdomains and ESCRT in ER stress-induced lipid droplet microautophagy in budding yeast.Mol Biol Cell. 2021 Dec 1;32(22):br12. doi: 10.1091/mbc.E21-04-0179. Epub 2021 Oct 20. Mol Biol Cell. 2021. PMID: 34668753 Free PMC article.
-
Yeast lipids can phase-separate into micrometer-scale membrane domains.J Biol Chem. 2010 Sep 24;285(39):30224-32. doi: 10.1074/jbc.M110.123554. Epub 2010 Jul 20. J Biol Chem. 2010. PMID: 20647309 Free PMC article.
-
Uptake and trafficking of exogenous sterols in Saccharomyces cerevisiae.Biochem Soc Trans. 2006 Jun;34(Pt 3):359-62. doi: 10.1042/BST0340359. Biochem Soc Trans. 2006. PMID: 16709161 Review.
-
How interaction of perfringolysin O with membranes is controlled by sterol structure, lipid structure, and physiological low pH: insights into the origin of perfringolysin O-lipid raft interaction.J Biol Chem. 2008 Feb 22;283(8):4632-42. doi: 10.1074/jbc.M709483200. Epub 2007 Dec 17. J Biol Chem. 2008. PMID: 18089559
Cited by
-
The Continuing Mystery of Lipid Rafts.J Mol Biol. 2016 Dec 4;428(24 Pt A):4749-4764. doi: 10.1016/j.jmb.2016.08.022. Epub 2016 Aug 26. J Mol Biol. 2016. PMID: 27575334 Free PMC article. Review.
-
Regimes of Complex Lipid Bilayer Phases Induced by Cholesterol Concentration in MD Simulation.Biophys J. 2018 Dec 4;115(11):2167-2178. doi: 10.1016/j.bpj.2018.10.011. Epub 2018 Oct 19. Biophys J. 2018. PMID: 30414630 Free PMC article.
-
Role of MCC/Eisosome in Fungal Lipid Homeostasis.Biomolecules. 2019 Jul 25;9(8):305. doi: 10.3390/biom9080305. Biomolecules. 2019. PMID: 31349700 Free PMC article. Review.
-
Using cyclodextrin-induced lipid substitution to study membrane lipid and ordered membrane domain (raft) function in cells.Biochim Biophys Acta Biomembr. 2022 Feb 1;1864(1):183774. doi: 10.1016/j.bbamem.2021.183774. Epub 2021 Sep 14. Biochim Biophys Acta Biomembr. 2022. PMID: 34534531 Free PMC article. Review.
-
Hallmarks of Reversible Separation of Living, Unperturbed Cell Membranes into Two Liquid Phases.Biophys J. 2017 Dec 5;113(11):2425-2432. doi: 10.1016/j.bpj.2017.09.029. Biophys J. 2017. PMID: 29211996 Free PMC article.
References
Publication types
MeSH terms
Substances
Grants and funding
LinkOut - more resources
Full Text Sources
Other Literature Sources
Molecular Biology Databases