Effects of phosphorylation on the structure and backbone dynamics of the intrinsically disordered connexin43 C-terminal domain
- PMID: 23828237
- PMCID: PMC3750180
- DOI: 10.1074/jbc.M113.454389
Effects of phosphorylation on the structure and backbone dynamics of the intrinsically disordered connexin43 C-terminal domain
Abstract
Phosphorylation of the connexin43 C-terminal (Cx43CT) domain regulates gap junction intercellular communication. However, an understanding of the mechanisms by which phosphorylation exerts its effects is lacking. Here, we test the hypothesis that phosphorylation regulates Cx43 gap junction intercellular communication by mediating structural changes in the C-terminal domain. Circular dichroism and nuclear magnetic resonance were used to characterize the effects of phosphorylation on the secondary structure and backbone dynamics of soluble and membrane-tethered Cx43CT domains. Cx43CT phospho-mimetic isoforms, which have Asp substitutions at specific Ser/Tyr sites, revealed phosphorylation alters the α-helical content of the Cx43CT domain only when attached to the membrane. The changes in secondary structure are due to variations in the conformational preference and backbone flexibility of residues adjacent and distal to the site(s) of modification. In addition to the known direct effects of phosphorylation on molecular partner interactions, the data presented here suggest phosphorylation may also indirectly regulate binding affinity by altering the conformational preference of the Cx43CT domain.
Keywords: Circular Dichroism (CD); Gap Junctions; Nuclear Magnetic Resonance; Phosphorylation; Protein Dynamics.
Figures
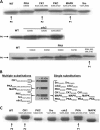
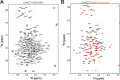
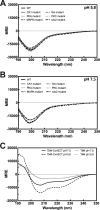
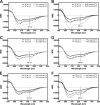
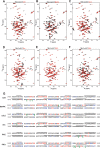
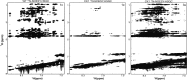
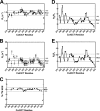
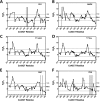
Similar articles
-
Structural changes in the carboxyl terminus of the gap junction protein connexin43 indicates signaling between binding domains for c-Src and zonula occludens-1.J Biol Chem. 2004 Dec 24;279(52):54695-701. doi: 10.1074/jbc.M409552200. Epub 2004 Oct 18. J Biol Chem. 2004. PMID: 15492000
-
Structural Studies of the Nedd4 WW Domains and Their Selectivity for the Connexin43 (Cx43) Carboxyl Terminus.J Biol Chem. 2016 Apr 1;291(14):7637-50. doi: 10.1074/jbc.M115.701417. Epub 2016 Feb 3. J Biol Chem. 2016. PMID: 26841867 Free PMC article.
-
Characterization of the pH-dependent interaction between the gap junction protein connexin43 carboxyl terminus and cytoplasmic loop domains.J Biol Chem. 2007 Feb 23;282(8):5801-13. doi: 10.1074/jbc.M605233200. Epub 2006 Dec 17. J Biol Chem. 2007. PMID: 17178730
-
Connexin43 phosphorylation: structural changes and biological effects.Biochem J. 2009 Apr 15;419(2):261-72. doi: 10.1042/BJ20082319. Biochem J. 2009. PMID: 19309313 Free PMC article. Review.
-
Structural bases for the chemical regulation of Connexin43 channels.Cardiovasc Res. 2004 May 1;62(2):268-75. doi: 10.1016/j.cardiores.2003.12.030. Cardiovasc Res. 2004. PMID: 15094347 Review.
Cited by
-
Roles of astrocytic connexin-43, hemichannels, and gap junctions in oxygen-glucose deprivation/reperfusion injury induced neuroinflammation and the possible regulatory mechanisms of salvianolic acid B and carbenoxolone.J Neuroinflammation. 2018 Mar 27;15(1):97. doi: 10.1186/s12974-018-1127-3. J Neuroinflammation. 2018. PMID: 29587860 Free PMC article.
-
The C-terminal domain of connexin43 modulates cartilage structure via chondrocyte phenotypic changes.Oncotarget. 2016 Nov 8;7(45):73055-73067. doi: 10.18632/oncotarget.12197. Oncotarget. 2016. PMID: 27682878 Free PMC article.
-
Structural determinants and proliferative consequences of connexin 37 hemichannel function in insulinoma cells.J Biol Chem. 2014 Oct 31;289(44):30379-30386. doi: 10.1074/jbc.M114.583054. Epub 2014 Sep 12. J Biol Chem. 2014. PMID: 25217644 Free PMC article.
-
Digested disorder: Quarterly intrinsic disorder digest (July-August-September, 2013).Intrinsically Disord Proteins. 2014 May 19;2(1):e27833. doi: 10.4161/idp.27833. eCollection 2014. Intrinsically Disord Proteins. 2014. PMID: 28232877 Free PMC article. Review.
-
Role of ROS/RNS in Preeclampsia: Are Connexins the Missing Piece?Int J Mol Sci. 2020 Jun 30;21(13):4698. doi: 10.3390/ijms21134698. Int J Mol Sci. 2020. PMID: 32630161 Free PMC article. Review.
References
-
- Kumar N. M., Gilula N. B. (1996) The gap junction communication channel. Cell 84, 381–388 - PubMed
-
- Laird D. W. (2008) Closing the gap on autosomal dominant connexin-26 and connexin-43 mutants linked to human disease. J. Biol. Chem. 283, 2997–3001 - PubMed
-
- Dobrowolski R., Willecke K. (2009) Connexin-caused genetic diseases and corresponding mouse models. Antioxid. Redox Signal. 11, 283–295 - PubMed
-
- Unger V. M., Kumar N. M., Gilula N. B., Yeager M. (1999) Three-dimensional structure of a recombinant gap junction membrane channel. Science 283, 1176–1180 - PubMed
-
- Maeda S., Nakagawa S., Suga M., Yamashita E., Oshima A., Fujiyoshi Y., Tsukihara T. (2009) Structure of the connexin 26 gap junction channel at 3.5 Å resolution. Nature 458, 597–602 - PubMed
Publication types
MeSH terms
Substances
Grants and funding
LinkOut - more resources
Full Text Sources
Other Literature Sources
Molecular Biology Databases
Miscellaneous