The secondary loss of gyrencephaly as an example of evolutionary phenotypical reversal
- PMID: 23805079
- PMCID: PMC3693069
- DOI: 10.3389/fnana.2013.00016
The secondary loss of gyrencephaly as an example of evolutionary phenotypical reversal
Abstract
Gyrencephaly (the folding of the surface of the neocortex) is a mammalian-specific trait present in almost all mammalian orders. Despite the widespread appearance of the trait, little is known about the mechanism of its genesis or its adaptive significance. Still, most of the hypotheses proposed concentrated on the pattern of connectivity of mature neurons as main components of gyri formation. Recent work on embryonic neurogenesis in several species of mammals revealed different progenitor and stem cells and their neurogenic potential as having important roles in the process of gyrification. Studies in the field of comparative neurogenesis revealed that gyrencephaly is an evolutionarily labile trait, and that some species underwent a secondary loss of a convoluted brain surface and thus reverted to a more ancient form, a less folded brain surface (lissencephaly). This phenotypic reversion provides an excellent system for understanding the phenomenon of secondary loss. In this review, we will outline the theory behind secondary loss and, as specific examples, present species that have undergone this transition with respect to neocortical folding. We will also discuss different possible pathways for obtaining (or losing) gyri. Finally, we will explore the potential adaptive consequence of gyrencephaly relative to lissencephaly and vice versa.
Keywords: brain evolution; gyrencephaly; lissencephaly; neocortex; reverse evolution.
Figures
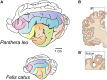
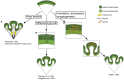
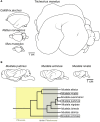
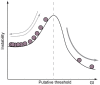
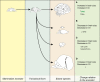
Similar articles
-
Conical expansion of the outer subventricular zone and the role of neocortical folding in evolution and development.Front Hum Neurosci. 2013 Aug 1;7:424. doi: 10.3389/fnhum.2013.00424. eCollection 2013. Front Hum Neurosci. 2013. PMID: 23914167 Free PMC article.
-
An adaptive threshold in mammalian neocortical evolution.PLoS Biol. 2014 Nov 18;12(11):e1002000. doi: 10.1371/journal.pbio.1002000. eCollection 2014 Nov. PLoS Biol. 2014. PMID: 25405475 Free PMC article.
-
Developing guinea pig brain as a model for cortical folding.Dev Growth Differ. 2017 May;59(4):286-301. doi: 10.1111/dgd.12371. Epub 2017 Jun 5. Dev Growth Differ. 2017. PMID: 28585227
-
Social Cues, Adult Neurogenesis, and Reproductive Behavior.In: Mucignat-Caretta C, editor. Neurobiology of Chemical Communication. Boca Raton (FL): CRC Press/Taylor & Francis; 2014. Chapter 13. In: Mucignat-Caretta C, editor. Neurobiology of Chemical Communication. Boca Raton (FL): CRC Press/Taylor & Francis; 2014. Chapter 13. PMID: 24830028 Free Books & Documents. Review.
-
Evolution of the mammalian dentate gyrus.J Comp Neurol. 2016 Feb 15;524(3):578-94. doi: 10.1002/cne.23851. Epub 2015 Jul 29. J Comp Neurol. 2016. PMID: 26179319 Free PMC article. Review.
Cited by
-
Microcephaly genes evolved adaptively throughout the evolution of eutherian mammals.BMC Evol Biol. 2014 Jun 5;14:120. doi: 10.1186/1471-2148-14-120. BMC Evol Biol. 2014. PMID: 24898820 Free PMC article.
-
Gyrification of the cerebral cortex requires FGF signaling in the mammalian brain.Elife. 2017 Nov 14;6:e29285. doi: 10.7554/eLife.29285. Elife. 2017. PMID: 29132503 Free PMC article.
-
A Digital Endocranial Cast of the Early Paleocene (Puercan) 'Archaic' Mammal Onychodectes tisonensis (Eutheria: Taeniodonta).J Mamm Evol. 2018;25(2):179-195. doi: 10.1007/s10914-017-9381-1. Epub 2017 Mar 7. J Mamm Evol. 2018. PMID: 29755252 Free PMC article.
-
Dynamic expression of calretinin in embryonic and early fetal human cortex.Front Neuroanat. 2014 Jun 3;8:41. doi: 10.3389/fnana.2014.00041. eCollection 2014. Front Neuroanat. 2014. PMID: 24917793 Free PMC article.
-
Resequencing of the TMF-1 (TATA Element Modulatory Factor) regulated protein (TRNP1) gene in domestic and wild canids.Canine Med Genet. 2023 Nov 15;10(1):10. doi: 10.1186/s40575-023-00133-0. Canine Med Genet. 2023. PMID: 37968761 Free PMC article.
References
-
- Barron D. H. (1950). An experimental analysis of some factors involved in the development of the fissure pattern of the cerebral cortex. J. Exp. Zool. 113, 553–581 10.1002/jez.1401130304 - DOI
-
- Cope E. (1896). The Primary Factors of Organic Evolution. Chicago: The Open Court Publishing Company
LinkOut - more resources
Full Text Sources
Other Literature Sources