Modelling the endothelial blood-CNS barriers: a method for the production of robust in vitro models of the rat blood-brain barrier and blood-spinal cord barrier
- PMID: 23773766
- PMCID: PMC3694476
- DOI: 10.1186/1471-2202-14-59
Modelling the endothelial blood-CNS barriers: a method for the production of robust in vitro models of the rat blood-brain barrier and blood-spinal cord barrier
Abstract
Background: Modelling the blood-CNS barriers of the brain and spinal cord in vitro continues to provide a considerable challenge for research studying the passage of large and small molecules in and out of the central nervous system, both within the context of basic biology and for pharmaceutical drug discovery. Although there has been considerable success over the previous two decades in establishing useful in vitro primary endothelial cell cultures from the blood-CNS barriers, no model fully mimics the high electrical resistance, low paracellular permeability and selective influx/efflux characteristics of the in vivo situation. Furthermore, such primary-derived cultures are typically labour-intensive and generate low yields of cells, limiting scope for experimental work. We thus aimed to establish protocols for the high yield isolation and culture of endothelial cells from both rat brain and spinal cord. Our aim was to optimise in vitro conditions for inducing phenotypic characteristics in these cells that were reminiscent of the in vivo situation, such that they developed into tight endothelial barriers suitable for performing investigative biology and permeability studies.
Methods: Brain and spinal cord tissue was taken from the same rats and used to specifically isolate endothelial cells to reconstitute as in vitro blood-CNS barrier models. Isolated endothelial cells were cultured to expand the cellular yield and then passaged onto cell culture inserts for further investigation. Cell culture conditions were optimised using commercially available reagents and the resulting barrier-forming endothelial monolayers were characterised by functional permeability experiments and in vitro phenotyping by immunocytochemistry and western blotting.
Results: Using a combination of modified handling techniques and cell culture conditions, we have established and optimised a protocol for the in vitro culture of brain and, for the first time in rat, spinal cord endothelial cells. High yields of both CNS endothelial cell types can be obtained, and these can be passaged onto large numbers of cell culture inserts for in vitro permeability studies. The passaged brain and spinal cord endothelial cells are pure and express endothelial markers, tight junction proteins and intracellular transport machinery. Further, both models exhibit tight, functional barrier characteristics that are discriminating against large and small molecules in permeability assays and show functional expression of the pharmaceutically important P-gp efflux transporter.
Conclusions: Our techniques allow the provision of high yields of robust sister cultures of endothelial cells that accurately model the blood-CNS barriers in vitro. These models are ideally suited for use in studying the biology of the blood-brain barrier and blood-spinal cord barrier in vitro and for pre-clinical drug discovery.
Figures
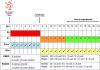
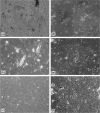
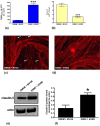
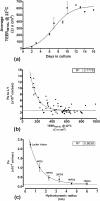
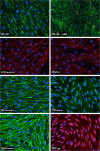
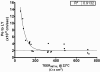
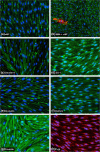
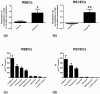
Similar articles
-
Setting-up an in vitro model of rat blood-brain barrier (BBB): a focus on BBB impermeability and receptor-mediated transport.J Vis Exp. 2014 Jun 28;(88):e51278. doi: 10.3791/51278. J Vis Exp. 2014. PMID: 24998179 Free PMC article.
-
In vitro models of the blood-brain barrier.Methods Mol Biol. 2014;1135:415-37. doi: 10.1007/978-1-4939-0320-7_34. Methods Mol Biol. 2014. PMID: 24510883
-
Modelling the neurovascular unit and the blood-brain barrier with the unique function of pericytes.Curr Neurovasc Res. 2011 Nov;8(4):258-69. doi: 10.2174/156720211798121016. Curr Neurovasc Res. 2011. PMID: 22023614
-
Rat brain endothelial cell lines for the study of blood-brain barrier permeability and transport functions.Cell Mol Neurobiol. 2005 Feb;25(1):41-58. doi: 10.1007/s10571-004-1376-9. Cell Mol Neurobiol. 2005. PMID: 15962508 Review.
-
Quantitative Proteomics-Based Blood-Brain Barrier Study.Biol Pharm Bull. 2021;44(4):465-473. doi: 10.1248/bpb.b21-00001. Biol Pharm Bull. 2021. PMID: 33790097 Review.
Cited by
-
In Vitro Blood-Brain Barrier Models for Neuroinfectious Diseases: A Narrative Review.Curr Neuropharmacol. 2024;22(8):1344-1373. doi: 10.2174/1570159X22666231207114346. Curr Neuropharmacol. 2024. PMID: 38073104 Free PMC article. Review.
-
Targeting brain cells with glutathione-modulated nanoliposomes: in vitro and in vivo study.Drug Des Devel Ther. 2015 Jul 20;9:3705-27. doi: 10.2147/DDDT.S85302. eCollection 2015. Drug Des Devel Ther. 2015. PMID: 26229435 Free PMC article.
-
Fabrication of Sealed Nanostraw Microdevices for Oral Drug Delivery.ACS Nano. 2016 Jun 28;10(6):5873-81. doi: 10.1021/acsnano.6b00809. Epub 2016 Jun 13. ACS Nano. 2016. PMID: 27268699 Free PMC article.
-
Drug Penetration into the Central Nervous System: Pharmacokinetic Concepts and In Vitro Model Systems.Pharmaceutics. 2021 Sep 23;13(10):1542. doi: 10.3390/pharmaceutics13101542. Pharmaceutics. 2021. PMID: 34683835 Free PMC article. Review.
-
Modeling the blood-brain barrier for treatment of central nervous system (CNS) diseases.J Tissue Eng. 2022 May 14;13:20417314221095997. doi: 10.1177/20417314221095997. eCollection 2022 Jan-Dec. J Tissue Eng. 2022. PMID: 35586265 Free PMC article. Review.
References
-
- Cecchelli R, Berezowski V, Lundquist S, Culot M, Renftel M, Dehouck MP, Fenart L. Modelling of the blood–brain barrier in drug discovery and development. Nat Rev Drug Discov. 2007;6(8):650–661. - PubMed
-
- Toth A, Veszelka S, Nakagawa S, Niwa M, Deli MA. Patented in vitro blood–brain barrier models in CNS drug discovery. Recent Pat CNS Drug Discov. 2011;6(2):107–118. - PubMed
-
- Zlokovic BV. The blood–brain barrier in health and chronic neurodegenerative disorders. Neuron. 2008;57(2):178–201. - PubMed
-
- Palmer AM. The role of the blood-CNS barrier in CNS disorders and their treatment. Neurobiol Dis. 2010;37(1):3–12. - PubMed
-
- Deli MA, Abraham CS, Kataoka Y, Niwa M. Permeability studies on in vitro blood–brain barrier models: physiology, pathology, and pharmacology. Cell Mol Neurobiol. 2005;25(1):59–127. - PubMed
MeSH terms
Substances
LinkOut - more resources
Full Text Sources
Other Literature Sources
Miscellaneous