Retinal input regulates the timing of corticogeniculate innervation
- PMID: 23761904
- PMCID: PMC3682386
- DOI: 10.1523/JNEUROSCI.5271-12.2013
Retinal input regulates the timing of corticogeniculate innervation
Abstract
Neurons in layer VI of visual cortex represent one of the largest sources of nonretinal input to the dorsal lateral geniculate nucleus (dLGN) and play a major role in modulating the gain of thalamic signal transmission. However, little is known about how and when these descending projections arrive and make functional connections with dLGN cells. Here we used a transgenic mouse to visualize corticogeniculate projections to examine the timing of cortical innervation in dLGN. Corticogeniculate innervation occurred at postnatal ages and was delayed compared with the arrival of retinal afferents. Cortical fibers began to enter dLGN at postnatal day 3 (P3) to P4, a time when retinogeniculate innervation is complete. However, cortical projections did not fully innervate dLGN until eye opening (P12), well after the time when retinal inputs from the two eyes segregate to form nonoverlapping eye-specific domains. In vitro thalamic slice recordings revealed that newly arriving cortical axons form functional connections with dLGN cells. However, adult-like responses that exhibited paired pulse facilitation did not fully emerge until 2 weeks of age. Finally, surgical or genetic elimination of retinal input greatly accelerated the rate of corticogeniculate innervation, with axons invading between P2 and P3 and fully innervating dLGN by P8 to P10. However, recordings in genetically deafferented mice showed that corticogeniculate synapses continued to mature at the same rate as controls. These studies suggest that retinal and cortical innervation of dLGN is highly coordinated and that input from retina plays an important role in regulating the rate of corticogeniculate innervation.
Figures
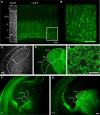
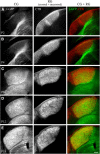
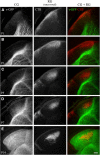
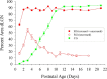
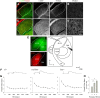
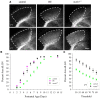
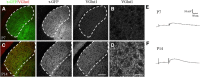
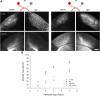
Similar articles
-
The absence of retinal input disrupts the development of cholinergic brainstem projections in the mouse dorsal lateral geniculate nucleus.Neural Dev. 2018 Dec 12;13(1):27. doi: 10.1186/s13064-018-0124-7. Neural Dev. 2018. PMID: 30541618 Free PMC article.
-
A molecular mechanism regulating the timing of corticogeniculate innervation.Cell Rep. 2013 Nov 14;5(3):573-81. doi: 10.1016/j.celrep.2013.09.041. Epub 2013 Oct 31. Cell Rep. 2013. PMID: 24183669 Free PMC article.
-
Reciprocal Connections Between Cortex and Thalamus Contribute to Retinal Axon Targeting to Dorsal Lateral Geniculate Nucleus.Cereb Cortex. 2018 Apr 1;28(4):1168-1182. doi: 10.1093/cercor/bhx028. Cereb Cortex. 2018. PMID: 28334242 Free PMC article.
-
How much feedback from visual cortex to lateral geniculate nucleus in cat: a perspective.Vis Neurosci. 2004 Jul-Aug;21(4):487-500. doi: 10.1017/S0952523804214018. Vis Neurosci. 2004. PMID: 15579216 Review.
-
Development of the mammalian retinogeniculate pathway: target finding, transient synapses and binocular segregation.J Exp Biol. 1990 Oct;153:85-104. doi: 10.1242/jeb.153.1.85. J Exp Biol. 1990. PMID: 2280230 Review.
Cited by
-
Wiring subcortical image-forming centers: Topography, laminar targeting, and map alignment.Curr Top Dev Biol. 2021;142:283-317. doi: 10.1016/bs.ctdb.2020.10.004. Epub 2020 Nov 16. Curr Top Dev Biol. 2021. PMID: 33706920 Free PMC article. Review.
-
The absence of retinal input disrupts the development of cholinergic brainstem projections in the mouse dorsal lateral geniculate nucleus.Neural Dev. 2018 Dec 12;13(1):27. doi: 10.1186/s13064-018-0124-7. Neural Dev. 2018. PMID: 30541618 Free PMC article.
-
Developmental Remodeling of Thalamic Interneurons Requires Retinal Signaling.J Neurosci. 2019 May 15;39(20):3856-3866. doi: 10.1523/JNEUROSCI.2224-18.2019. Epub 2019 Mar 6. J Neurosci. 2019. PMID: 30842249 Free PMC article.
-
Neuronal and Synaptic Plasticity in the Visual Thalamus in Mouse Models of Glaucoma.Front Cell Neurosci. 2021 Jan 15;14:626056. doi: 10.3389/fncel.2020.626056. eCollection 2020. Front Cell Neurosci. 2021. PMID: 33584206 Free PMC article.
-
Development of Functional Properties in the Early Visual System: New Appreciations of the Roles of Lateral Geniculate Nucleus.Curr Top Behav Neurosci. 2022;53:3-35. doi: 10.1007/7854_2021_297. Curr Top Behav Neurosci. 2022. PMID: 35112333 Review.
References
Publication types
MeSH terms
Substances
Grants and funding
LinkOut - more resources
Full Text Sources
Other Literature Sources
Molecular Biology Databases
Miscellaneous