Visualizing GroEL/ES in the act of encapsulating a folding protein
- PMID: 23746846
- PMCID: PMC3695626
- DOI: 10.1016/j.cell.2013.04.052
Visualizing GroEL/ES in the act of encapsulating a folding protein
Abstract
The GroEL/ES chaperonin system is required for the assisted folding of many proteins. How these substrate proteins are encapsulated within the GroEL-GroES cavity is poorly understood. Using symmetry-free, single-particle cryo-electron microscopy, we have characterized a chemically modified mutant of GroEL (EL43Py) that is trapped at a normally transient stage of substrate protein encapsulation. We show that the symmetric pattern of the GroEL subunits is broken as the GroEL cis-ring apical domains reorient to accommodate the simultaneous binding of GroES and an incompletely folded substrate protein (RuBisCO). The collapsed RuBisCO folding intermediate binds to the lower segment of two apical domains, as well as to the normally unstructured GroEL C-terminal tails. A comparative structural analysis suggests that the allosteric transitions leading to substrate protein release and folding involve concerted shifts of GroES and the GroEL apical domains and C-terminal tails.
Copyright © 2013 Elsevier Inc. All rights reserved.
Figures
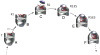
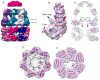
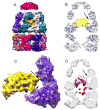
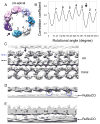
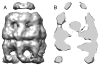
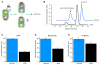
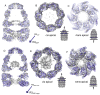
Similar articles
-
Efficient Catalysis of Protein Folding by GroEL/ES of the Obligate Chaperonin Substrate MetF.J Mol Biol. 2020 Mar 27;432(7):2304-2318. doi: 10.1016/j.jmb.2020.02.031. Epub 2020 Mar 2. J Mol Biol. 2020. PMID: 32135190
-
Revisiting the GroEL-GroES reaction cycle via the symmetric intermediate implied by novel aspects of the GroEL(D398A) mutant.J Biol Chem. 2008 Aug 29;283(35):23774-81. doi: 10.1074/jbc.M802542200. Epub 2008 Jun 20. J Biol Chem. 2008. PMID: 18567584 Free PMC article.
-
TEM and STEM-EDS evaluation of metal nanoparticle encapsulation in GroEL/GroES complexes according to the reaction mechanism of chaperonin.Microscopy (Oxf). 2021 Jun 6;70(3):289-296. doi: 10.1093/jmicro/dfaa064. Microscopy (Oxf). 2021. PMID: 33173948
-
GroEL assisted folding of large polypeptide substrates in Escherichia coli: Present scenario and assignments for the future.Prog Biophys Mol Biol. 2009 Jan;99(1):42-50. doi: 10.1016/j.pbiomolbio.2008.10.007. Epub 2008 Nov 7. Prog Biophys Mol Biol. 2009. PMID: 19027782 Review.
-
GroEL-assisted protein folding: does it occur within the chaperonin inner cavity?Int J Mol Sci. 2009 May 12;10(5):2066-2083. doi: 10.3390/ijms10052066. Int J Mol Sci. 2009. PMID: 19564940 Free PMC article. Review.
Cited by
-
Protein chain collapse modulation and folding stimulation by GroEL-ES.Sci Adv. 2022 Mar 4;8(9):eabl6293. doi: 10.1126/sciadv.abl6293. Epub 2022 Mar 4. Sci Adv. 2022. PMID: 35245117 Free PMC article.
-
MitCHAP-60 and Hereditary Spastic Paraplegia SPG-13 Arise from an Inactive hsp60 Chaperonin that Fails to Fold the ATP Synthase β-Subunit.Sci Rep. 2019 Aug 23;9(1):12300. doi: 10.1038/s41598-019-48762-5. Sci Rep. 2019. PMID: 31444388 Free PMC article.
-
Chaperone machines for protein folding, unfolding and disaggregation.Nat Rev Mol Cell Biol. 2013 Oct;14(10):630-42. doi: 10.1038/nrm3658. Epub 2013 Sep 12. Nat Rev Mol Cell Biol. 2013. PMID: 24026055 Free PMC article. Review.
-
Mechanisms and pathology of protein misfolding and aggregation.Nat Rev Mol Cell Biol. 2023 Dec;24(12):912-933. doi: 10.1038/s41580-023-00647-2. Epub 2023 Sep 8. Nat Rev Mol Cell Biol. 2023. PMID: 37684425 Review.
-
Novel cryo-EM structure of an ADP-bound GroEL-GroES complex.Sci Rep. 2021 Sep 14;11(1):18241. doi: 10.1038/s41598-021-97657-x. Sci Rep. 2021. PMID: 34521893 Free PMC article.
References
-
- Badcoe IG, Smith CJ, Wood S, Halsall DJ, Holbrook JJ, Lund P, Clarke AR. Binding of a chaperonin to the folding intermediates of lactate dehydrogenase. Biochemistry. 1991;30:9195–9200. - PubMed
-
- Braig K, Otwinowski Z, Hegde R, Boisvert D, Joachimiak A, Horwich A, Sigler P. The crystal structure of the bacterial chaperonin GroEL at 2.8 A. Nature. 1994;371:578–586. - PubMed
-
- Burston SG, Ranson NA, Clarke AR. The origins and consequences of asymmetry in the chaperonin reaction cycle. J Mol Biol. 1995;249:138–152. - PubMed
-
- Chen D, Song J, Chuang D, Chiu W, Ludtke S. An expanded conformation of single-ring GroEL-GroES complex encapsulates an 86 kDa substrate. Structure. 2006;14:1711–1722. - PubMed
Publication types
MeSH terms
Substances
Associated data
- Actions
- Actions
- Actions
Grants and funding
LinkOut - more resources
Full Text Sources
Other Literature Sources
Molecular Biology Databases
Research Materials