Entry of enveloped viruses into host cells: membrane fusion
- PMID: 23737062
- PMCID: PMC7121288
- DOI: 10.1007/978-94-007-6552-8_16
Entry of enveloped viruses into host cells: membrane fusion
Abstract
Viruses are intracellular parasites that hijack the cellular machinery for their own replication. Therefore, an obligatory step in the virus life cycle is the delivery of the viral genome inside the cell. Enveloped viruses (i.e., viruses with a lipid envelope) use a two-step procedure to release their genetic material into the cell: (i) they first bind to specific surface receptors of the target cell membrane and then, (ii) they fuse the viral and cell membranes. This last step may occur at the cell surface or after internalization of the virus particle by endocytosis or by some other route (e.g., macropinocytosis). Remarkably, the virus-cell membrane fusion process goes essentially along the same intermediate steps as other membrane fusions that occur for instance in vesicular fusion at the nerve synapsis or cell-cell fusion in yeast mating. Specialized viral proteins, fusogens, promote virus-cell membrane fusion. The viral fusogens experience drastic structural rearrangements during fusion, liberating the energy required to overcome the repulsive forces that prevent spontaneous fusion of the two membranes. This chapter describes the different types of viral fusogens and their mode of action, as are currently known.
Figures
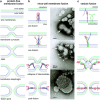
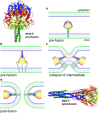
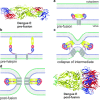
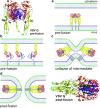
Similar articles
-
Entry of Enveloped Viruses into Host Cells: Membrane Fusion.Subcell Biochem. 2024;105:567-592. doi: 10.1007/978-3-031-65187-8_16. Subcell Biochem. 2024. PMID: 39738958 Review.
-
The Art of Viral Membrane Fusion and Penetration.Subcell Biochem. 2023;106:113-152. doi: 10.1007/978-3-031-40086-5_4. Subcell Biochem. 2023. PMID: 38159225
-
Virus-Mediated Cell-Cell Fusion.Int J Mol Sci. 2020 Dec 17;21(24):9644. doi: 10.3390/ijms21249644. Int J Mol Sci. 2020. PMID: 33348900 Free PMC article. Review.
-
Roles of Cholesterol in Early and Late Steps of the Nipah Virus Membrane Fusion Cascade.J Virol. 2021 Feb 24;95(6):e02323-20. doi: 10.1128/JVI.02323-20. Print 2021 Feb 24. J Virol. 2021. PMID: 33408170 Free PMC article.
-
Membrane fusion and fission: enveloped viruses.Protein Pept Lett. 2009;16(7):751-9. doi: 10.2174/092986609788681760. Protein Pept Lett. 2009. PMID: 19601904 Review.
Cited by
-
Antiviral Activity of the Rhamnolipids Mixture from the Antarctic Bacterium Pseudomonas gessardii M15 against Herpes Simplex Viruses and Coronaviruses.Pharmaceutics. 2021 Dec 8;13(12):2121. doi: 10.3390/pharmaceutics13122121. Pharmaceutics. 2021. PMID: 34959400 Free PMC article.
-
Microcystis viridis NIES-102 Cyanobacteria Lectin (MVL) Interacts with SARS-CoV-2 Spike Protein Receptor Binding Domains (RBDs) via Protein-Protein Interaction.Int J Mol Sci. 2024 Jun 18;25(12):6696. doi: 10.3390/ijms25126696. Int J Mol Sci. 2024. PMID: 38928400 Free PMC article.
-
Herpes Simplex Virus: The Hostile Guest That Takes Over Your Home.Front Microbiol. 2020 May 7;11:733. doi: 10.3389/fmicb.2020.00733. eCollection 2020. Front Microbiol. 2020. PMID: 32457704 Free PMC article. Review.
-
Antigenic and sequence variability of the human respiratory syncytial virus F glycoprotein compared to related viruses in a comprehensive dataset.Vaccine. 2018 Oct 29;36(45):6660-6673. doi: 10.1016/j.vaccine.2018.09.056. Epub 2018 Oct 3. Vaccine. 2018. PMID: 30292456 Free PMC article.
-
Virucidal Activity of the Pyridobenzothiazolone Derivative HeE1-17Y against Enveloped RNA Viruses.Viruses. 2022 May 27;14(6):1157. doi: 10.3390/v14061157. Viruses. 2022. PMID: 35746629 Free PMC article.
References
References and Further Reading
Further Reading
-
- Palfreyman MT, Jorgensen EM (2009) In vivo analysis of membrane fusion. In: Encyclopedia of Life Sciences (ELS). John Wiley & Sons, Chichester. doi:10.1002/9780470015902.a0020891
-
- Helenius A. Virus entry and uncoating. In: Knipe DM, Howley PM, editors. Fields virology. 5. Philadelphia: Lippincott, Williams and Wilkins; 2007.
-
- Also especially recommended for further reading are references [4, 9, 15, 42, 44] listed above
Publication types
MeSH terms
LinkOut - more resources
Full Text Sources
Other Literature Sources