Cell migration
- PMID: 23720251
- PMCID: PMC4457291
- DOI: 10.1002/cphy.c110012
Cell migration
Abstract
Cell migration is fundamental to establishing and maintaining the proper organization of multicellular organisms. Morphogenesis can be viewed as a consequence, in part, of cell locomotion, from large-scale migrations of epithelial sheets during gastrulation, to the movement of individual cells during development of the nervous system. In an adult organism, cell migration is essential for proper immune response, wound repair, and tissue homeostasis, while aberrant cell migration is found in various pathologies. Indeed, as our knowledge of migration increases, we can look forward to, for example, abating the spread of highly malignant cancer cells, retarding the invasion of white cells in the inflammatory process, or enhancing the healing of wounds. This article is organized in two main sections. The first section is devoted to the single-cell migrating in isolation such as occurs when leukocytes migrate during the immune response or when fibroblasts squeeze through connective tissue. The second section is devoted to cells collectively migrating as part of multicellular clusters or sheets. This second type of migration is prevalent in development, wound healing, and in some forms of cancer metastasis.
© 2012 American Physiological Society
Figures
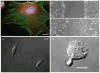
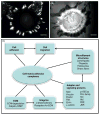
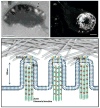
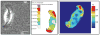
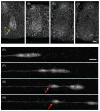
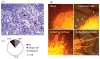
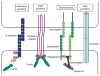
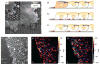
Similar articles
-
Cell adhesion and its endocytic regulation in cell migration during neural development and cancer metastasis.Int J Mol Sci. 2012;13(4):4564-4590. doi: 10.3390/ijms13044564. Epub 2012 Apr 11. Int J Mol Sci. 2012. PMID: 22605996 Free PMC article. Review.
-
E-cadherin plays an essential role in collective directional migration of large epithelial sheets.Cell Mol Life Sci. 2012 Aug;69(16):2779-89. doi: 10.1007/s00018-012-0951-3. Epub 2012 Mar 13. Cell Mol Life Sci. 2012. PMID: 22410739 Free PMC article.
-
The connexin mimetic peptide Gap27 increases human dermal fibroblast migration in hyperglycemic and hyperinsulinemic conditions in vitro.J Cell Physiol. 2012 Jan;227(1):77-87. doi: 10.1002/jcp.22705. Epub 2011 Feb 24. J Cell Physiol. 2012. PMID: 21984074
-
Modeling keratinocyte wound healing dynamics: Cell-cell adhesion promotes sustained collective migration.J Theor Biol. 2016 Jul 7;400:103-17. doi: 10.1016/j.jtbi.2016.04.015. Epub 2016 Apr 19. J Theor Biol. 2016. PMID: 27105673 Free PMC article.
-
PI3K and RAC signalling in leukocyte and cancer cell migration.Bull Cancer. 2006 May;93(5):E44-52. Bull Cancer. 2006. PMID: 16777617 Review.
Cited by
-
Accelerating Cell Migration along Radially Aligned Nanofibers through the Addition of Electrosprayed Nanoparticles in a Radial Density Gradient.Part Part Syst Charact. 2022 Apr;39(4):2100280. doi: 10.1002/ppsc.202100280. Epub 2022 Feb 26. Part Part Syst Charact. 2022. PMID: 36091327 Free PMC article.
-
Comparative profiling of cellular gait on adhesive micropatterns defines statistical patterns of activity that underlie native and cancerous cell dynamics.bioRxiv [Preprint]. 2023 Oct 27:2023.10.27.564389. doi: 10.1101/2023.10.27.564389. bioRxiv. 2023. PMID: 37961146 Free PMC article. Preprint.
-
Small body size of pseudoscorpions and a distinct architecture of the ovary: A step to miniaturization?J Anat. 2021 Nov;239(5):1182-1195. doi: 10.1111/joa.13485. Epub 2021 Jun 15. J Anat. 2021. PMID: 34131910 Free PMC article.
-
Increased migration of olfactory ensheathing cells secreting the Nogo receptor ectodomain over inhibitory substrates and lesioned spinal cord.Cell Mol Life Sci. 2015 Jul;72(14):2719-37. doi: 10.1007/s00018-015-1869-3. Epub 2015 Feb 24. Cell Mol Life Sci. 2015. PMID: 25708702 Free PMC article.
-
HHIP's Dynamic Role in Epithelial Wound Healing Reveals a Potential Mechanism of COPD Susceptibility.bioRxiv [Preprint]. 2024 Oct 8:2024.09.05.611545. doi: 10.1101/2024.09.05.611545. bioRxiv. 2024. PMID: 39416045 Free PMC article. Preprint.
References
-
- Abercrombie M, Heaysman JE, Pegrum SM. The locomotion of fibroblasts in culture. 3. Movements of particles on the dorsal surface of the leading lamella. Exp Cell Res. 1970;62:389–398. - PubMed
-
- Aberle H, Butz S, Stappert J, Weissig H, Kemler R, Hoschuetzky H. Assembly of the cadherin-catenin complex in vitro with recombinant proteins. J Cell Sci. 1994;107(Pt 12):3655–3663. - PubMed
-
- Abrams EW, Vining MS, Andrew DJ. Constructing an organ: The Drosophila salivary gland as a model for tube formation. Trends Cell Biol. 2003;13:247–254. - PubMed
-
- Agarwal R, D’Souza T, Morin PJ. Claudin-3 and claudin-4 expression in ovarian epithelial cells enhances invasion and is associated with increased matrix metalloproteinase-2 activity. Cancer Res. 2005;65:7378–7385. - PubMed
Publication types
MeSH terms
Grants and funding
LinkOut - more resources
Full Text Sources