N-glycosylation of ICAM-2 is required for ICAM-2-mediated complete suppression of metastatic potential of SK-N-AS neuroblastoma cells
- PMID: 23714211
- PMCID: PMC3700849
- DOI: 10.1186/1471-2407-13-261
N-glycosylation of ICAM-2 is required for ICAM-2-mediated complete suppression of metastatic potential of SK-N-AS neuroblastoma cells
Abstract
Background: Cell adhesion molecules (CAMs) are expressed ubiquitously. Each of the four families of CAMs is comprised of glycosylated, membrane-bound proteins that participate in multiple cellular processes including cell-cell communication, cell motility, inside-out and outside-in signaling, tumorigenesis, angiogenesis and metastasis. Intercellular adhesion molecule-2 (ICAM-2), a member of the immunoglobulin superfamily of CAMs, has six N-linked glycosylation sites at amino acids (asparagines) 47, 82, 105, 153, 178 and 187. Recently, we demonstrated a previously unknown function for ICAM-2 in tumor cells. We showed that ICAM-2 suppressed neuroblastoma cell motility and growth in soft agar, and induced a juxtamembrane distribution of F-actin in vitro. We also showed that ICAM-2 completely suppressed development of disseminated tumors in vivo in a murine model of metastatic NB. These effects of ICAM-2 on NB cell phenotype in vitro and in vivo depended on the interaction of ICAM-2 with the cytoskeletal linker protein α-actinin. Interestingly, ICAM-2 did not suppress subcutaneous growth of tumors in mice, suggesting that ICAM-2 affects the metastatic but not the tumorigenic potential of NB cells. The goal of the study presented here was to determine if the glycosylation status of ICAM-2 influenced its function in neuroblastoma cells.
Methods: Because it is well documented that glycosylation facilitates essential steps in tumor progression and metastasis, we investigated whether the glycosylation status of ICAM-2 affected the phenotype of NB cells. We used site-directed mutagenesis to express hypo- or non-glycosylated variants of ICAM-2, by substituting alanine for asparagine at glycosylation sites, and compared the impact of each variant on NB cell motility, anchorage-independent growth, interaction with intracellular proteins, effect on F-actin distribution and metastatic potential in vivo.
Results: The in vitro and in vivo phenotypes of cells expressing glycosylation site variants differed from cells expressing fully-glycosylated ICAM-2 or no ICAM-2. Most striking was the finding that mice injected intravenously with NB cells expressing glycosylation site variants survived longer (P ≤ 0.002) than mice receiving SK-N-AS cells with undetectable ICAM-2. However, unlike fully-glycosylated ICAM-2, glycosylation site variants did not completely suppress disseminated tumor development.
Conclusions: Reduced glycosylation of ICAM-2 significantly attenuated, but did not abolish, its ability to suppress metastatic properties of NB cells.
Figures
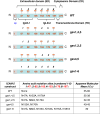
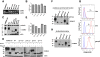
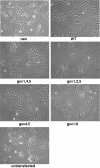
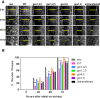
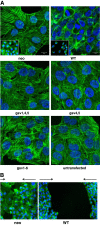
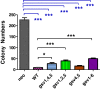
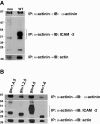
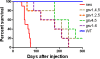
Similar articles
-
ICAM-2 confers a non-metastatic phenotype in neuroblastoma cells by interaction with α-actinin.Oncogene. 2015 Mar 19;34(12):1553-62. doi: 10.1038/onc.2014.87. Epub 2014 Apr 7. Oncogene. 2015. PMID: 24704826 Free PMC article.
-
ICAM-2 expression mediates a membrane-actin link, confers a nonmetastatic phenotype and reflects favorable tumor stage or histology in neuroblastoma.PLoS One. 2008;3(11):e3629. doi: 10.1371/journal.pone.0003629. Epub 2008 Nov 3. PLoS One. 2008. PMID: 18978946 Free PMC article.
-
Binding of the cytoplasmic domain of intercellular adhesion molecule-2 (ICAM-2) to alpha-actinin.J Biol Chem. 1996 Oct 18;271(42):26214-9. doi: 10.1074/jbc.271.42.26214. J Biol Chem. 1996. PMID: 8824270
-
Cell adhesion molecules as targets for therapy of neuroblastoma.Cancer Biol Ther. 2009 Feb;8(4):306-11. doi: 10.4161/cbt.8.4.7446. Epub 2009 Feb 19. Cancer Biol Ther. 2009. PMID: 19197150 Review.
-
An overview of neuroblastoma cell lineage phenotypes and in vitro models.Exp Biol Med (Maywood). 2020 Dec;245(18):1637-1647. doi: 10.1177/1535370220949237. Epub 2020 Aug 12. Exp Biol Med (Maywood). 2020. PMID: 32787463 Free PMC article. Review.
Cited by
-
The Extracellular Matrix and Neuroblastoma Cell Communication-A Complex Interplay and Its Therapeutic Implications.Cells. 2022 Oct 10;11(19):3172. doi: 10.3390/cells11193172. Cells. 2022. PMID: 36231134 Free PMC article. Review.
-
The role of ICAM-2 in neuroblastoma.Oncoscience. 2015 Nov 23;2(11):915-6. doi: 10.18632/oncoscience.273. eCollection 2015. Oncoscience. 2015. PMID: 26697524 Free PMC article. No abstract available.
-
Serum Protein N-Glycosylation Signatures of Neuroblastoma.Front Oncol. 2021 Mar 16;11:603417. doi: 10.3389/fonc.2021.603417. eCollection 2021. Front Oncol. 2021. PMID: 33796450 Free PMC article.
-
Identification and characterization of the intercellular adhesion molecule-2 gene as a novel p53 target.Oncotarget. 2016 Sep 20;7(38):61426-61437. doi: 10.18632/oncotarget.11366. Oncotarget. 2016. PMID: 27556181 Free PMC article.
-
TRPM7 maintains progenitor-like features of neuroblastoma cells: implications for metastasis formation.Oncotarget. 2015 Apr 20;6(11):8760-76. doi: 10.18632/oncotarget.3315. Oncotarget. 2015. PMID: 25797249 Free PMC article.
References
-
- Leda J, Yokoyama S, Tamura K, Takifuji K, Hotta T, Matsuda K, Oku Y, Nasu T, Kiriyama S, Yamamoto N, Nakamura Y, Shively JE, Yamaue H. Re-expression of CEACAM1 long cytoplasmic domain isoform is associated with invasion and migration of colorectal cancer. Int J Cancer. 2011;129(6):1351–1361. doi: 10.1002/ijc.26072. - DOI - PubMed
Publication types
MeSH terms
Substances
Grants and funding
LinkOut - more resources
Full Text Sources
Other Literature Sources
Medical
Research Materials
Miscellaneous