Aurora B spatially regulates EB3 phosphorylation to coordinate daughter cell adhesion with cytokinesis
- PMID: 23712260
- PMCID: PMC3664705
- DOI: 10.1083/jcb.201301131
Aurora B spatially regulates EB3 phosphorylation to coordinate daughter cell adhesion with cytokinesis
Abstract
During mitosis, human cells round up, decreasing their adhesion to extracellular substrates. This must be quickly reestablished by poorly understood cytoskeleton remodeling mechanisms that prevent detachment from epithelia, while ensuring the successful completion of cytokinesis. Here we show that the microtubule end-binding (EB) proteins EB1 and EB3 play temporally distinct roles throughout cell division. Whereas EB1 was involved in spindle orientation before anaphase, EB3 was required for stabilization of focal adhesions and coordinated daughter cell spreading during mitotic exit. Additionally, EB3 promoted midbody microtubule stability and, consequently, midbody stabilization necessary for efficient cytokinesis. Importantly, daughter cell adhesion and cytokinesis completion were spatially regulated by distinct states of EB3 phosphorylation on serine 176 by Aurora B. This EB3 phosphorylation was enriched at the midbody and shown to control cortical microtubule growth. These findings uncover differential roles of EB proteins and explain the importance of an Aurora B phosphorylation gradient for the spatiotemporal regulation of microtubule function during mitotic exit and cytokinesis.
Figures
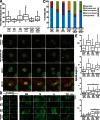
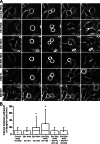
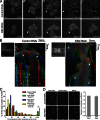
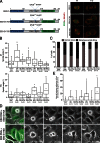
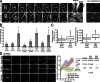
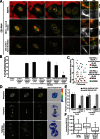
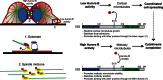
Similar articles
-
Phosphorylation of ZEN-4/MKLP1 by aurora B regulates completion of cytokinesis.Curr Biol. 2005 Apr 26;15(8):778-86. doi: 10.1016/j.cub.2005.03.041. Curr Biol. 2005. PMID: 15854913
-
Aurora B interaction of centrosomal Nlp regulates cytokinesis.J Biol Chem. 2010 Dec 17;285(51):40230-9. doi: 10.1074/jbc.M110.140541. Epub 2010 Sep 23. J Biol Chem. 2010. PMID: 20864540 Free PMC article.
-
EB1 promotes Aurora-B kinase activity through blocking its inactivation by protein phosphatase 2A.Proc Natl Acad Sci U S A. 2008 May 20;105(20):7153-8. doi: 10.1073/pnas.0710018105. Epub 2008 May 13. Proc Natl Acad Sci U S A. 2008. PMID: 18477699 Free PMC article.
-
Late mitotic functions of Aurora kinases.Chromosoma. 2017 Feb;126(1):93-103. doi: 10.1007/s00412-016-0594-5. Epub 2016 Apr 22. Chromosoma. 2017. PMID: 27106516 Review.
-
Chromosomal passengers: the four-dimensional regulation of mitotic events.Chromosoma. 2004 Nov;113(5):211-22. doi: 10.1007/s00412-004-0307-3. Epub 2004 Sep 4. Chromosoma. 2004. PMID: 15351889 Review.
Cited by
-
Microtubule plus-ends act as physical signaling hubs to activate RhoA during cytokinesis.Elife. 2019 Feb 13;8:e38968. doi: 10.7554/eLife.38968. Elife. 2019. PMID: 30758285 Free PMC article.
-
Helder Maiato: hot (+)TIPs on mitosis. Interview by Caitlin Sedwick.J Cell Biol. 2013 Sep 2;202(5):722-3. doi: 10.1083/jcb.2025pi. J Cell Biol. 2013. PMID: 23999165 Free PMC article.
-
The microtubule-associated protein EB1 links AIM2 inflammasomes with autophagy-dependent secretion.J Biol Chem. 2014 Oct 17;289(42):29322-33. doi: 10.1074/jbc.M114.559153. Epub 2014 Aug 27. J Biol Chem. 2014. PMID: 25164813 Free PMC article.
-
α-Tubulin detyrosination links the suppression of MCAK activity with taxol cytotoxicity.J Cell Biol. 2023 Feb 6;222(2):e202205092. doi: 10.1083/jcb.202205092. Epub 2022 Dec 2. J Cell Biol. 2023. PMID: 36459065 Free PMC article.
-
A proteomic study of mitotic phase-specific interactors of EB1 reveals a role for SXIP-mediated protein interactions in anaphase onset.Biol Open. 2015 Jan 16;4(2):155-69. doi: 10.1242/bio.201410413. Biol Open. 2015. PMID: 25596275 Free PMC article.
References
-
- Ban R., Matsuzaki H., Akashi T., Sakashita G., Taniguchi H., Park S.Y., Tanaka H., Furukawa K., Urano T. 2009. Mitotic regulation of the stability of microtubule plus-end tracking protein EB3 by ubiquitin ligase SIAH-1 and Aurora mitotic kinases. J. Biol. Chem. 284:28367–28381 10.1074/jbc.M109.000273 - DOI - PMC - PubMed
Publication types
MeSH terms
Substances
LinkOut - more resources
Full Text Sources
Other Literature Sources
Molecular Biology Databases
Miscellaneous