Improving the thermal, radial, and temporal accuracy of the analytical ultracentrifuge through external references
- PMID: 23711724
- PMCID: PMC3826449
- DOI: 10.1016/j.ab.2013.05.011
Improving the thermal, radial, and temporal accuracy of the analytical ultracentrifuge through external references
Abstract
Sedimentation velocity (SV) is a method based on first principles that provides a precise hydrodynamic characterization of macromolecules in solution. Due to recent improvements in data analysis, the accuracy of experimental SV data emerges as a limiting factor in its interpretation. Our goal was to unravel the sources of experimental error and develop improved calibration procedures. We implemented the use of a Thermochron iButton temperature logger to directly measure the temperature of a spinning rotor and detected deviations that can translate into an error of as much as 10% in the sedimentation coefficient. We further designed a precision mask with equidistant markers to correct for instrumental errors in the radial calibration that were observed to span a range of 8.6%. The need for an independent time calibration emerged with use of the current data acquisition software (Zhao et al., Anal. Biochem., 437 (2013) 104-108), and we now show that smaller but significant time errors of up to 2% also occur with earlier versions. After application of these calibration corrections, the sedimentation coefficients obtained from 11 instruments displayed a significantly reduced standard deviation of approximately 0.7%. This study demonstrates the need for external calibration procedures and regular control experiments with a sedimentation coefficient standard.
Keywords: Hydrodynamic modeling; Sedimentation equilibrium; Sedimentation velocity.
Published by Elsevier Inc.
Figures
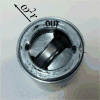
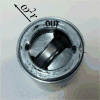
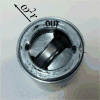
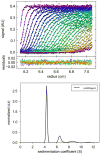
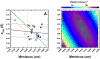
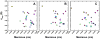
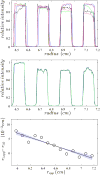
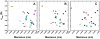
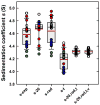
Similar articles
-
A multilaboratory comparison of calibration accuracy and the performance of external references in analytical ultracentrifugation.PLoS One. 2015 May 21;10(5):e0126420. doi: 10.1371/journal.pone.0126420. eCollection 2015. PLoS One. 2015. PMID: 25997164 Free PMC article.
-
Improved measurement of the rotor temperature in analytical ultracentrifugation.Anal Biochem. 2014 Apr 15;451:69-75. doi: 10.1016/j.ab.2014.02.006. Epub 2014 Feb 14. Anal Biochem. 2014. PMID: 24530285 Free PMC article.
-
A radial calibration window for analytical ultracentrifugation.PLoS One. 2018 Jul 30;13(7):e0201529. doi: 10.1371/journal.pone.0201529. eCollection 2018. PLoS One. 2018. PMID: 30059530 Free PMC article.
-
Analytical ultracentrifugation for the study of protein association and assembly.Curr Opin Chem Biol. 2006 Oct;10(5):430-6. doi: 10.1016/j.cbpa.2006.08.017. Epub 2006 Aug 28. Curr Opin Chem Biol. 2006. PMID: 16935549 Review.
-
Modern analytical ultracentrifugation in protein science: a tutorial review.Protein Sci. 2002 Sep;11(9):2067-79. doi: 10.1110/ps.0207702. Protein Sci. 2002. PMID: 12192063 Free PMC article. Review.
Cited by
-
Quantitative Analysis of Protein Self-Association by Sedimentation Velocity.Curr Protoc Protein Sci. 2020 Sep;101(1):e109. doi: 10.1002/cpps.109. Curr Protoc Protein Sci. 2020. PMID: 32614509 Free PMC article.
-
'AND' logic gates at work: Crystal structure of Rad53 bound to Dbf4 and Cdc7.Sci Rep. 2016 Sep 29;6:34237. doi: 10.1038/srep34237. Sci Rep. 2016. PMID: 27681475 Free PMC article.
-
Measuring aggregates, self-association, and weak interactions in concentrated therapeutic antibody solutions.MAbs. 2020 Jan-Dec;12(1):1810488. doi: 10.1080/19420862.2020.1810488. MAbs. 2020. PMID: 32887536 Free PMC article.
-
Tools for the quantitative analysis of sedimentation boundaries detected by fluorescence optical analytical ultracentrifugation.PLoS One. 2013 Oct 18;8(10):e77245. doi: 10.1371/journal.pone.0077245. eCollection 2013. PLoS One. 2013. PMID: 24204779 Free PMC article.
-
Variable Field Analytical Ultracentrifugation: II. Gravitational Sweep Sedimentation Velocity.Biophys J. 2016 Jan 5;110(1):103-12. doi: 10.1016/j.bpj.2015.11.027. Biophys J. 2016. PMID: 26745414 Free PMC article.
References
-
- Howlett GJ, Minton AP, Rivas G. Analytical ultracentrifugation for the study of protein association and assembly. Curr Opin Chem Biol. 2006;10:430–436. - PubMed
-
- Schuck P, Zhao H. Editorial for the special issue of methods “Modern Analytical Ultracentrifugation”. Methods. 2011;54:1–3. - PubMed
-
- Arisaka F. Applications and future perspectives of analytical ultracentrifugation. Tanpakushitsu Kakusan Koso. 1999;44:82–91. - PubMed
-
- Harding SE, Rowe AJ. Insight into protein-protein interactions from analytical ultracentrifugation. Biochem Soc Transact. 2010;38:901–7. - PubMed
-
- Trautman R. The impact of the desk-top computer on ultracentrifugation. Ann N Y Acad Sci. 1969;164:52–65.
Publication types
MeSH terms
Substances
Grants and funding
LinkOut - more resources
Full Text Sources
Other Literature Sources