Proton channel HVCN1 is required for effector functions of mouse eosinophils
- PMID: 23705768
- PMCID: PMC3668235
- DOI: 10.1186/1471-2172-14-24
Proton channel HVCN1 is required for effector functions of mouse eosinophils
Abstract
Background: Proton currents are required for optimal respiratory burst in phagocytes. Recently, HVCN1 was identified as the molecule required for the voltage-gated proton channel activity associated with the respiratory burst in neutrophils. Although there are similarities between eosinophils and neutrophils regarding their mechanism for respiratory burst, the role of proton channels in eosinophil functions has not been fully understood.
Results: In the present study, we first identified the expression of the proton channel HVCN1 in mouse eosinophils. Furthermore, using HVCN1-deficient eosinophils, we demonstrated important cell-specific effector functions for HVCN1. Similar to HVCN1-deficient neutrophils, HVCN1-deficient eosinophils produced significantly less reactive oxygen species (ROS) upon phorbol myristate acetate (PMA) stimulation compared with WT eosinophils. In contrast to HVCN1-deficient neutrophils, HVCN1-deficient eosinophils did not show impaired calcium mobilization or migration ability compared with wild-type (WT) cells. Uniquely, HVCN1-deficient eosinophils underwent significantly increased cell death induced by PMA stimulation compared with WT eosinophils. The increased cell death was dependent on NADPH oxidase activation, and correlated with the failure of HVCN1-deficient cells to maintain membrane polarization and intracellular pH in the physiological range upon activation.
Conclusions: Eosinophils require proton channel HVCN1 for optimal ROS generation and prevention of activation-induced cell death.
Figures
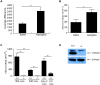
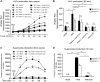
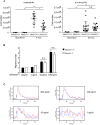
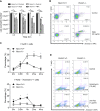
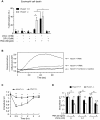
Similar articles
-
Identification of Thr29 as a critical phosphorylation site that activates the human proton channel Hvcn1 in leukocytes.J Biol Chem. 2010 Feb 19;285(8):5117-21. doi: 10.1074/jbc.C109.082727. Epub 2009 Dec 26. J Biol Chem. 2010. PMID: 20037153 Free PMC article.
-
Comparison of proton channel, phagocyte oxidase, and respiratory burst levels between human eosinophil and neutrophil granulocytes.Free Radic Res. 2014 Oct;48(10):1190-9. doi: 10.3109/10715762.2014.938234. Epub 2014 Jul 21. Free Radic Res. 2014. PMID: 24985354
-
Sustained activation of proton channels and NADPH oxidase in human eosinophils and murine granulocytes requires PKC but not cPLA2 alpha activity.J Physiol. 2007 Mar 1;579(Pt 2):327-44. doi: 10.1113/jphysiol.2006.124248. Epub 2006 Dec 21. J Physiol. 2007. PMID: 17185330 Free PMC article.
-
The intimate and controversial relationship between voltage-gated proton channels and the phagocyte NADPH oxidase.Immunol Rev. 2016 Sep;273(1):194-218. doi: 10.1111/imr.12437. Immunol Rev. 2016. PMID: 27558336 Free PMC article. Review.
-
pH regulation and beyond: unanticipated functions for the voltage-gated proton channel, HVCN1.Trends Cell Biol. 2011 Jan;21(1):20-8. doi: 10.1016/j.tcb.2010.09.006. Epub 2010 Oct 18. Trends Cell Biol. 2011. PMID: 20961760 Free PMC article. Review.
Cited by
-
The Voltage-Gated Proton Channel: A Riddle, Wrapped in a Mystery, inside an Enigma.Biochemistry. 2015 Jun 2;54(21):3250-68. doi: 10.1021/acs.biochem.5b00353. Epub 2015 May 20. Biochemistry. 2015. PMID: 25964989 Free PMC article. Review.
-
Zinc modulation of proton currents in a new voltage-gated proton channel suggests a mechanism of inhibition.FEBS J. 2020 Nov;287(22):4996-5018. doi: 10.1111/febs.15291. Epub 2020 Apr 6. FEBS J. 2020. PMID: 32160407 Free PMC article.
-
Voltage and pH sensing by the voltage-gated proton channel, HV1.J R Soc Interface. 2018 Apr;15(141):20180108. doi: 10.1098/rsif.2018.0108. J R Soc Interface. 2018. PMID: 29643227 Free PMC article. Review.
-
Voltage-Gated Proton Channels as Novel Drug Targets: From NADPH Oxidase Regulation to Sperm Biology.Antioxid Redox Signal. 2015 Aug 10;23(5):490-513. doi: 10.1089/ars.2013.5806. Epub 2014 Mar 17. Antioxid Redox Signal. 2015. PMID: 24483328 Free PMC article. Review.
-
Role of voltage-gated proton channel (Hv1) in cancer biology.Front Pharmacol. 2023 Apr 20;14:1175702. doi: 10.3389/fphar.2023.1175702. eCollection 2023. Front Pharmacol. 2023. PMID: 37153807 Free PMC article. Review.
References
-
- DeLeo FR, Quinn MT. Assembly of the phagocyte NADPH oxidase: molecular interaction of oxidase proteins. J Leukoc Biol. 1996;60:677–691. - PubMed
MeSH terms
Substances
Grants and funding
LinkOut - more resources
Full Text Sources
Other Literature Sources