Insights into the Molecular Mechanisms Underlying Mammalian P2X7 Receptor Functions and Contributions in Diseases, Revealed by Structural Modeling and Single Nucleotide Polymorphisms
- PMID: 23675347
- PMCID: PMC3646254
- DOI: 10.3389/fphar.2013.00055
Insights into the Molecular Mechanisms Underlying Mammalian P2X7 Receptor Functions and Contributions in Diseases, Revealed by Structural Modeling and Single Nucleotide Polymorphisms
Abstract
The mammalian P2X7 receptors (P2X7Rs), a member of the ionotropic P2X receptor family with distinctive functional properties, play an important part in mediating extracellular ATP signaling in health and disease. A clear delineation of the molecular mechanisms underlying the key receptor properties, such as ATP-binding, ion permeation, and large pore formation of the mammalian P2X7Rs, is still lacking, but such knowledge is crucial for a better understanding of their physiological functions and contributions in diseases and for development of therapeutics. The recent breakthroughs in determining the atomic structures of the zebrafish P2X4.1R in the closed and ATP-bound open states have provided the long-awaited structural information. The human P2RX7 gene is abundant with non-synonymous single nucleotide polymorphisms (NS-SNPs), which generate a repertoire of human P2X7Rs with point mutations. Characterizations of the NS-SNPs identified in patients of various disease conditions and the resulting mutations have informed previously unknown molecular mechanisms determining the mammalian P2X7R functions and diseases. In this review, we will discuss the new insights into such mechanisms provided by structural modeling and recent functional and genetic linkage studies of NS-SNPs.
Keywords: ATP-binding; NS-SNPs; P2X7R; extracellular ATP; ion channel; large pore; structural modeling.
Figures
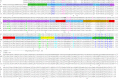
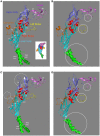
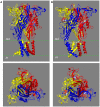
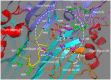
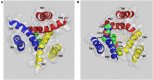
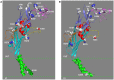
Similar articles
-
Single nucleotide polymorphisms that were identified in affective mood disorders affect ATP-activated P2X7 receptor functions.J Psychiatr Res. 2010 Apr;44(6):347-55. doi: 10.1016/j.jpsychires.2009.10.005. Epub 2009 Nov 20. J Psychiatr Res. 2010. PMID: 19931869
-
P2X7 receptors: channels, pores and more.CNS Neurol Disord Drug Targets. 2012 Sep;11(6):705-21. doi: 10.2174/187152712803581137. CNS Neurol Disord Drug Targets. 2012. PMID: 22963440 Review.
-
Non-synonymous single nucleotide polymorphisms in the P2X receptor genes: association with diseases, impact on receptor functions and potential use as diagnosis biomarkers.Int J Mol Sci. 2014 Jul 30;15(8):13344-71. doi: 10.3390/ijms150813344. Int J Mol Sci. 2014. PMID: 25079442 Free PMC article. Review.
-
Localization of the gate and selectivity filter of the full-length P2X7 receptor.Proc Natl Acad Sci U S A. 2017 Mar 14;114(11):E2156-E2165. doi: 10.1073/pnas.1610414114. Epub 2017 Feb 24. Proc Natl Acad Sci U S A. 2017. PMID: 28235784 Free PMC article.
-
Functional P2X7 Receptors in the Auditory Nerve of Hearing Rodents Localize Exclusively to Peripheral Glia.J Neurosci. 2021 Mar 24;41(12):2615-2629. doi: 10.1523/JNEUROSCI.2240-20.2021. Epub 2021 Feb 9. J Neurosci. 2021. PMID: 33563723 Free PMC article.
Cited by
-
Trophic activity of human P2X7 receptor isoforms A and B in osteosarcoma.PLoS One. 2014 Sep 16;9(9):e107224. doi: 10.1371/journal.pone.0107224. eCollection 2014. PLoS One. 2014. PMID: 25226385 Free PMC article.
-
Hypothalamic and ovarian transcriptome profiling reveals potential candidate genes in low and high egg production of white Muscovy ducks (Cairina moschata).Poult Sci. 2021 Sep;100(9):101310. doi: 10.1016/j.psj.2021.101310. Epub 2021 Jun 6. Poult Sci. 2021. PMID: 34298381 Free PMC article.
-
The P2X7 Receptor in Osteoarthritis.Front Cell Dev Biol. 2021 Feb 11;9:628330. doi: 10.3389/fcell.2021.628330. eCollection 2021. Front Cell Dev Biol. 2021. PMID: 33644066 Free PMC article. Review.
-
Z1456467176 alleviates gouty arthritis by allosterically modulating P2X7R to inhibit NLRP3 inflammasome activation.Front Pharmacol. 2022 Aug 16;13:979939. doi: 10.3389/fphar.2022.979939. eCollection 2022. Front Pharmacol. 2022. PMID: 36052144 Free PMC article.
-
P2X7 Receptors in Neurodegeneration: Potential Therapeutic Applications From Basic to Clinical Approaches.Front Cell Neurosci. 2021 Apr 6;15:617036. doi: 10.3389/fncel.2021.617036. eCollection 2021. Front Cell Neurosci. 2021. PMID: 33889073 Free PMC article. Review.
References
-
- Adinolfi E., Callegari M. G., Ferrari D., Bolognesi C., Minelli M., Wieckowski M. R., et al. (2005). Basal activation of the P2X7 ATP receptor elevates mitochondrial calcium and potential, increases cellular ATP levels, and promotes serum-independent growth. Mol. Biol. Cell 16, 3260–327210.1091/mbc.E04-11-1025 - DOI - PMC - PubMed
LinkOut - more resources
Full Text Sources
Other Literature Sources
Miscellaneous