Conformational properties of peptides corresponding to the ebolavirus GP2 membrane-proximal external region in the presence of micelle-forming surfactants and lipids
- PMID: 23650881
- PMCID: PMC3772975
- DOI: 10.1021/bi400040v
Conformational properties of peptides corresponding to the ebolavirus GP2 membrane-proximal external region in the presence of micelle-forming surfactants and lipids
Abstract
Ebola virus and Sudan virus are members of the family Filoviridae of nonsegmented negative-strand RNA viruses ("filoviruses") that cause severe hemorrhagic fever with fatality rates as high as 90%. Infection by filoviruses requires membrane fusion between the host and the virus; this process is facilitated by the two subunits of the envelope glycoprotein, GP1 (the surface subunit) and GP2 (the transmembrane subunit). The membrane-proximal external region (MPER) is a Trp-rich segment that immediately precedes the transmembrane domain of GP2. In the analogous glycoprotein for HIV-1 (gp41), the MPER is critical for membrane fusion and is the target of several neutralizing antibodies. However, the role of the MPER in filovirus GP2 and its importance in membrane fusion have not been established. Here, we characterize the conformational properties of peptides representing the GP MPER segments of Ebola virus and Sudan virus in the presence of micelle-forming surfactants and lipids, at pH 7 and 4.6. Circular dichroism spectroscopy and tryptophan fluorescence indicate that the GP2 MPER peptides bind to micelles of sodium dodecyl sulfate and dodecylphosphocholine (DPC). Nuclear magnetic resonance spectroscopy of the Sudan virus MPER peptide revealed that residues 644-651 interact directly with DPC, and that this interaction enhances the helical conformation of the peptide. The Sudan virus MPER peptide was found to moderately inhibit cell entry by a GP-pseudotyped vesicular stomatitis virus but did not induce leakage of a fluorescent molecule from a large unilammellar vesicle comprised of 1-palmitoyl-2-oleoylphosphatidylcholine or cause hemolysis. Taken together, this analysis suggests the filovirus GP2 MPER binds and inserts shallowly into lipid membranes.
Conflict of interest statement
The authors declare no competing financial interest.
Figures
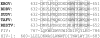
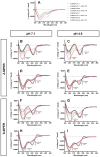
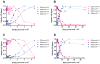
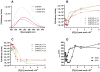
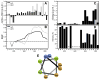
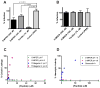
Similar articles
-
Structure of the Ebola virus envelope protein MPER/TM domain and its interaction with the fusion loop explains their fusion activity.Proc Natl Acad Sci U S A. 2017 Sep 19;114(38):E7987-E7996. doi: 10.1073/pnas.1708052114. Epub 2017 Sep 5. Proc Natl Acad Sci U S A. 2017. PMID: 28874543 Free PMC article.
-
Functional importance of the coiled-coil of the Ebola virus glycoprotein.J Virol. 2000 Nov;74(21):10194-201. doi: 10.1128/jvi.74.21.10194-10201.2000. J Virol. 2000. PMID: 11024148 Free PMC article.
-
Crystal structure of the Marburg virus GP2 core domain in its postfusion conformation.Biochemistry. 2012 Oct 2;51(39):7665-75. doi: 10.1021/bi300976m. Epub 2012 Sep 19. Biochemistry. 2012. PMID: 22935026 Free PMC article.
-
NMR Structure of the FIV gp36 C-Terminal Heptad Repeat and Membrane-Proximal External Region.Int J Mol Sci. 2020 Mar 16;21(6):2037. doi: 10.3390/ijms21062037. Int J Mol Sci. 2020. PMID: 32188158 Free PMC article.
-
The membrane-proximal tryptophan-rich region of the HIV glycoprotein, gp41, forms a well-defined helix in dodecylphosphocholine micelles.Biochemistry. 2001 Aug 14;40(32):9570-8. doi: 10.1021/bi010640u. Biochemistry. 2001. PMID: 11583156
Cited by
-
Conformational and lipid bilayer-perturbing properties of Marburg virus GP2 segments containing the fusion loop and membrane-proximal external region/transmembrane domain.Heliyon. 2019 Dec 12;5(12):e03018. doi: 10.1016/j.heliyon.2019.e03018. eCollection 2019 Dec. Heliyon. 2019. PMID: 31890962 Free PMC article.
-
A Review: The Antiviral Activity of Cyclic Peptides.Int J Pept Res Ther. 2023;29(1):7. doi: 10.1007/s10989-022-10478-y. Epub 2022 Dec 1. Int J Pept Res Ther. 2023. PMID: 36471676 Free PMC article. Review.
-
Structure of the Ebola virus envelope protein MPER/TM domain and its interaction with the fusion loop explains their fusion activity.Proc Natl Acad Sci U S A. 2017 Sep 19;114(38):E7987-E7996. doi: 10.1073/pnas.1708052114. Epub 2017 Sep 5. Proc Natl Acad Sci U S A. 2017. PMID: 28874543 Free PMC article.
-
Conditional trimerization and lytic activity of HIV-1 gp41 variants containing the membrane-associated segments.Biochemistry. 2015 Mar 3;54(8):1589-99. doi: 10.1021/bi501376f. Epub 2015 Feb 13. Biochemistry. 2015. PMID: 25658332 Free PMC article.
-
Membranotropic peptides mediating viral entry.Pept Sci (Hoboken). 2018 Sep;110(5):e24040. doi: 10.1002/pep2.24040. Epub 2018 Feb 13. Pept Sci (Hoboken). 2018. PMID: 32328541 Free PMC article. Review.
References
-
- Kuhn JH, Bao Y, Bavari S, Becker S, Bradfute S, Brister JR, Bukreyev AA, Chandran K, Davey RA, Dolnik O, Dye JM, Enterlein S, Hensley LE, Honko AN, Jahrling PB, Johnson KM, Kobinger G, Leroy EM, Lever MS, Mühlberger E, Netesov SV, Olinger GG, Palacios G, Patterson JL, Paweska JT, Pitt L, Radoshitzky SR, Saphire EO, Smither SJ, Swanepoel R, Towner JS, van der Groen G, Volchkov VE, Wahl-Jensen V, Warren TK, Weidmann M, Nichol ST. Virus nomenclature below the species level: a standardized nomenclature for natural variants of viruses assigned to the family Filoviridae. Arch Virol. 2012;155:2083–2103. - PMC - PubMed
-
- Sanchez A, Kiley MP, Holloway BP, Auperin DD. Sequence analysis of the Ebola virus genome: organization, genetic elements, and comparison with the genome of Marburg virus. Virus Res. 1993;29:215–240. - PubMed
Publication types
MeSH terms
Substances
Grants and funding
LinkOut - more resources
Full Text Sources
Other Literature Sources
Medical