Thalamic microcircuits: presynaptic dendrites form two feedforward inhibitory pathways in thalamus
- PMID: 23615551
- PMCID: PMC3727071
- DOI: 10.1152/jn.00559.2012
Thalamic microcircuits: presynaptic dendrites form two feedforward inhibitory pathways in thalamus
Abstract
In the visual thalamus, retinal afferents activate both local interneurons and excitatory thalamocortical relay neurons, leading to robust feedforward inhibition that regulates the transmission of sensory information from retina to neocortex. Peculiarly, this feedforward inhibitory pathway is dominated by presynaptic dendrites. Previous work has shown that the output of dendritic terminals of interneurons, also known as F2 terminals, are regulated by both ionotropic and metabotropic glutamate receptors. However, it is unclear whether both classes of glutamate receptors regulate output from the same or distinct dendritic terminals. Here, we used focal glutamate uncaging and whole cell recordings to reveal two types of F2 responses in rat visual thalamus. The first response, which we are calling a Type-A response, was mediated exclusively by ionotropic glutamate receptors (i.e., AMPA and NMDA). In contrast, the second response, which we are calling a Type-B response, was mediated by a combination of ionotropic and type 5 metabotropic glutamate receptors (i.e., mGluR(5)). In addition, we demonstrate that both F2 responses are evoked in the same postsynaptic neurons, which are morphologically distinct from neurons in which no F2 responses are observed. Since photostimulation was relatively focal and small in magnitude, these results suggest distinct F2 terminals, or small clusters of terminals, could be responsible for generating the two inhibitory responses observed. Because of the nature of ionotropic and metabotropic glutamate receptors, we predict the efficacy by which the retina communicates with the thalamus would be strongly regulated by 1) the activity level of a given retinogeniculate axon, and 2) the specific type of F2 terminals activated.
Keywords: dorsal lateral geniculate nucleus; glutamate uncaging; interneuron; presynaptic dendrite; two-photon imaging.
Figures
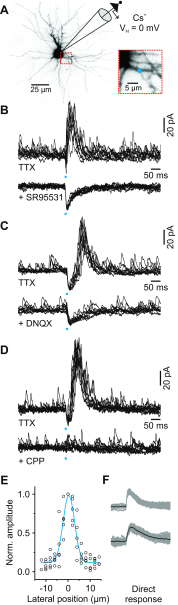
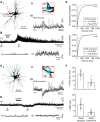
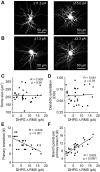
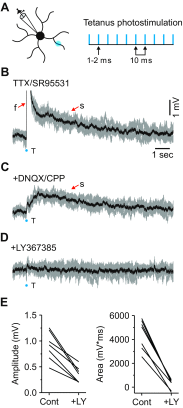
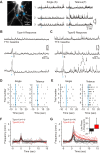
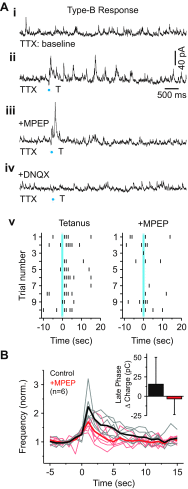
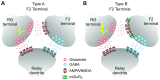
Similar articles
-
The multifaceted role of inhibitory interneurons in the dorsal lateral geniculate nucleus.Vis Neurosci. 2017 Jan;34:E017. doi: 10.1017/S0952523817000141. Vis Neurosci. 2017. PMID: 28965520 Review.
-
Distinct kinetics of inhibitory currents in thalamocortical neurons that arise from dendritic or axonal origin.PLoS One. 2017 Dec 18;12(12):e0189690. doi: 10.1371/journal.pone.0189690. eCollection 2017. PLoS One. 2017. PMID: 29252999 Free PMC article.
-
Control of dendritic outputs of inhibitory interneurons in the lateral geniculate nucleus.Neuron. 2000 Sep;27(3):597-610. doi: 10.1016/s0896-6273(00)00069-6. Neuron. 2000. PMID: 11055441
-
Synaptic activation of metabotropic glutamate receptors regulates dendritic outputs of thalamic interneurons.Neuron. 2004 Feb 19;41(4):611-23. doi: 10.1016/s0896-6273(04)00013-3. Neuron. 2004. PMID: 14980209
-
Complex regulation of dendritic transmitter release from thalamic interneurons.Curr Opin Neurobiol. 2014 Dec;29:126-32. doi: 10.1016/j.conb.2014.07.004. Epub 2014 Jul 23. Curr Opin Neurobiol. 2014. PMID: 25062503 Free PMC article. Review.
Cited by
-
Acetylcholine-dependent upregulation of TASK-1 channels in thalamic interneurons by a smooth muscle-like signalling pathway.J Physiol. 2017 Sep 1;595(17):5875-5893. doi: 10.1113/JP274527. Epub 2017 Aug 3. J Physiol. 2017. PMID: 28714121 Free PMC article.
-
Refinement of Spatial Receptive Fields in the Developing Mouse Lateral Geniculate Nucleus Is Coordinated with Excitatory and Inhibitory Remodeling.J Neurosci. 2018 May 9;38(19):4531-4542. doi: 10.1523/JNEUROSCI.2857-17.2018. Epub 2018 Apr 16. J Neurosci. 2018. PMID: 29661964 Free PMC article.
-
Thalamic neuromodulation and its implications for executive networks.Front Neural Circuits. 2014 Jun 24;8:69. doi: 10.3389/fncir.2014.00069. eCollection 2014. Front Neural Circuits. 2014. PMID: 25009467 Free PMC article. Review.
-
The subcellular distribution of T-type Ca2+ channels in interneurons of the lateral geniculate nucleus.PLoS One. 2014 Sep 30;9(9):e107780. doi: 10.1371/journal.pone.0107780. eCollection 2014. PLoS One. 2014. PMID: 25268996 Free PMC article.
-
Individual thalamic inhibitory interneurons are functionally specialized toward distinct visual features.Neuron. 2024 Aug 21;112(16):2765-2782.e9. doi: 10.1016/j.neuron.2024.06.001. Epub 2024 Jun 24. Neuron. 2024. PMID: 38917805 Free PMC article.
References
Publication types
MeSH terms
Substances
Grants and funding
LinkOut - more resources
Full Text Sources
Other Literature Sources
Miscellaneous