The in vivo TRPV6 protein starts at a non-AUG triplet, decoded as methionine, upstream of canonical initiation at AUG
- PMID: 23612980
- PMCID: PMC3675598
- DOI: 10.1074/jbc.M113.469726
The in vivo TRPV6 protein starts at a non-AUG triplet, decoded as methionine, upstream of canonical initiation at AUG
Abstract
TRPV6 channels function as epithelial Ca(2+) entry pathways in the epididymis, prostate, and placenta. However, the identity of the endogenous TRPV6 protein relies on predicted gene coding regions and is only known to a certain level of approximation. We show that in vivo the TRPV6 protein has an extended N terminus. Translation initiates at a non-AUG codon, at ACG, which is decoded by methionine and which is upstream of the annotated AUG, which is not used for initiation. The in vitro properties of channels formed by the extended full-length TRPV6 proteins and the so-far annotated and smaller TRPV6 are similar, but the extended N terminus increases trafficking to the plasma membrane and represents an additional scaffold for channel assembly. The increased translation of the smaller TRPV6 cDNA version may overestimate the in vivo situation where translation efficiency may represent an additional mechanism to tightly control the TRPV6-mediated Ca(2+) entry to prevent deleterious Ca(2+) overload.
Keywords: Antibodies; Calcium Channels; Mass Spectrometry (MS); TRP Channels; TRPV6; Translation; Translation Initiation at Non-AUG.
Figures
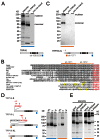
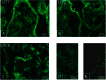
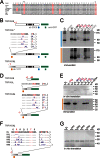
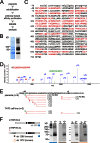
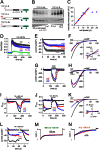
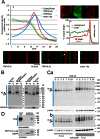
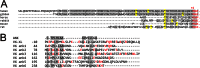
Similar articles
-
TRPV6 channels.Handb Exp Pharmacol. 2014;222:359-84. doi: 10.1007/978-3-642-54215-2_14. Handb Exp Pharmacol. 2014. PMID: 24756713 Review.
-
Why endogenous TRPV6 currents are not detectable-what can we learn from bats?Cell Calcium. 2020 Dec;92:102302. doi: 10.1016/j.ceca.2020.102302. Epub 2020 Oct 6. Cell Calcium. 2020. PMID: 33069057
-
Canonical transient receptor potential (TRPC) 1 acts as a negative regulator for vanilloid TRPV6-mediated Ca2+ influx.J Biol Chem. 2012 Oct 12;287(42):35612-35620. doi: 10.1074/jbc.M112.400952. Epub 2012 Aug 29. J Biol Chem. 2012. PMID: 22932896 Free PMC article.
-
The transient receptor potential cation channel subfamily V member 6 (TRPV6): genetics, biochemical properties, and functions of exceptional calcium channel proteins.Biochem Cell Biol. 2014 Dec;92(6):441-8. doi: 10.1139/bcb-2014-0063. Epub 2014 Aug 26. Biochem Cell Biol. 2014. PMID: 25372600 Review.
-
TRPV6.Handb Exp Pharmacol. 2007;(179):221-34. doi: 10.1007/978-3-540-34891-7_13. Handb Exp Pharmacol. 2007. PMID: 17217060 Review.
Cited by
-
Coupling of TRPV6 and TMEM16A in epithelial principal cells of the rat epididymis.J Gen Physiol. 2016 Aug;148(2):161-82. doi: 10.1085/jgp.201611626. J Gen Physiol. 2016. PMID: 27481714 Free PMC article.
-
Comparative proteomics reveals different protein expression in platelets in patients with alcoholic liver cirrhosis.Proteome Sci. 2024 Jan 26;22(1):3. doi: 10.1186/s12953-024-00227-y. Proteome Sci. 2024. PMID: 38279183 Free PMC article.
-
Mitotic Maturation Compensates for Premature Centrosome Splitting and PCM Loss in Human cep135 Knockout Cells.Cells. 2022 Apr 1;11(7):1189. doi: 10.3390/cells11071189. Cells. 2022. PMID: 35406752 Free PMC article.
-
Outside the limit: questioning the distance restrictions for cooperative miRNA binding sites.Cell Mol Biol Lett. 2023 Jan 24;28(1):8. doi: 10.1186/s11658-023-00421-4. Cell Mol Biol Lett. 2023. PMID: 36694129 Free PMC article.
-
TRPV6 Regulation by Cis-22a and Cholesterol.Biomolecules. 2022 Jun 8;12(6):804. doi: 10.3390/biom12060804. Biomolecules. 2022. PMID: 35740929 Free PMC article.
References
-
- Peng J. B., Chen X. Z., Berger U. V., Vassilev P. M., Tsukaguchi H., Brown E. M., Hediger M. A. (1999) Molecular cloning and characterization of a channel-like transporter mediating intestinal calcium absorption. J. Biol. Chem. 274, 22739–22746 - PubMed
-
- Peng J. B., Chen X. Z., Berger U. V., Weremowicz S., Morton C. C., Vassilev P. M., Brown E. M., Hediger M. A. (2000) Human calcium transport protein CaT1. Biochem. Biophys. Res. Commun. 278, 326–332 - PubMed
-
- Wissenbach U., Niemeyer B. A., Fixemer T., Schneidewind A., Trost C., Cavalie A., Reus K., Meese E., Bonkhoff H., Flockerzi V. (2001) Expression of CaT-like, a novel calcium-selective channel, correlates with the malignancy of prostate cancer. J. Biol. Chem. 276, 19461–19468 - PubMed
-
- Weissgerber P., Kriebs U., Tsvilovskyy V., Olausson J., Kretz O., Stoerger C., Mannebach S., Wissenbach U., Vennekens R., Middendorff R., Flockerzi V., Freichel M. (2012) Excision of Trpv6 gene leads to severe defects in epididymal Ca2+ absorption and male fertility much like single D541A pore mutation. J. Biol. Chem. 287, 17930–17941 - PMC - PubMed
Publication types
MeSH terms
Substances
LinkOut - more resources
Full Text Sources
Other Literature Sources
Molecular Biology Databases
Miscellaneous