Transient formation of water-conducting states in membrane transporters
- PMID: 23610412
- PMCID: PMC3651479
- DOI: 10.1073/pnas.1218986110
Transient formation of water-conducting states in membrane transporters
Abstract
Membrane transporters rely on highly coordinated structural transitions between major conformational states for their function, to prevent simultaneous access of the substrate binding site to both sides of the membrane--a mode of operation known as the alternating access model. Although this mechanism successfully accounts for the efficient exchange of the primary substrate across the membrane, accruing evidence on significant water transport and even uncoupled ion transport mediated by transporters has challenged the concept of perfect mechanical coupling and coordination of the gating mechanism in transporters, which might be expected from the alternating access model. Here, we present a large set of extended equilibrium molecular dynamics simulations performed on several classes of membrane transporters in different conformational states, to test the presence of the phenomenon in diverse transporter classes and to investigate the underlying molecular mechanism of water transport through membrane transporters. The simulations reveal spontaneous formation of transient water-conducting (channel-like) states allowing passive water diffusion through the lumen of the transporters. These channel-like states are permeable to water but occluded to substrate, thereby not hindering the uphill transport of the primary substrate, i.e., the alternating access model remains applicable to the substrate. The rise of such water-conducting states during the large-scale structural transitions of the transporter protein is indicative of imperfections in the coordinated closing and opening motions of the cytoplasmic and extracellular gates. We propose that the observed water-conducting states likely represent a universal phenomenon in membrane transporters, which is consistent with their reliance on large-scale motion for function.
Keywords: ABC transporters; LeuT-fold transporters; major facilitator superfamily; neurotransmitter transporters.
Conflict of interest statement
The authors declare no conflict of interest.
Figures
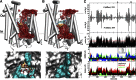
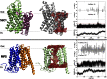
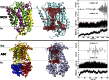
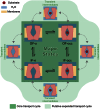
Similar articles
-
Locking Two Rigid-body Bundles in an Outward-Facing Conformation: The Ion-coupling Mechanism in a LeuT-fold Transporter.Sci Rep. 2019 Dec 20;9(1):19479. doi: 10.1038/s41598-019-55722-6. Sci Rep. 2019. PMID: 31862903 Free PMC article.
-
Structural elements required for coupling ion and substrate transport in the neurotransmitter transporter homolog LeuT.Proc Natl Acad Sci U S A. 2018 Sep 18;115(38):E8854-E8862. doi: 10.1073/pnas.1716870115. Epub 2018 Sep 4. Proc Natl Acad Sci U S A. 2018. PMID: 30181291 Free PMC article.
-
Microscopic Characterization of Membrane Transporter Function by In Silico Modeling and Simulation.Methods Enzymol. 2016;578:373-428. doi: 10.1016/bs.mie.2016.05.042. Epub 2016 Jul 11. Methods Enzymol. 2016. PMID: 27497175 Free PMC article. Review.
-
Identification of a second substrate-binding site in solute-sodium symporters.J Biol Chem. 2015 Jan 2;290(1):127-41. doi: 10.1074/jbc.M114.584383. Epub 2014 Nov 14. J Biol Chem. 2015. PMID: 25398883 Free PMC article.
-
Mechanistic aspects of sodium-binding sites in LeuT-like fold symporters.Biol Chem. 2013 May;394(5):641-8. doi: 10.1515/hsz-2012-0336. Biol Chem. 2013. PMID: 23362203 Review.
Cited by
-
Molecular Dynamics Simulation for All.Neuron. 2018 Sep 19;99(6):1129-1143. doi: 10.1016/j.neuron.2018.08.011. Neuron. 2018. PMID: 30236283 Free PMC article. Review.
-
Multiscale Simulations of Biological Membranes: The Challenge To Understand Biological Phenomena in a Living Substance.Chem Rev. 2019 May 8;119(9):5607-5774. doi: 10.1021/acs.chemrev.8b00538. Epub 2019 Mar 12. Chem Rev. 2019. PMID: 30859819 Free PMC article.
-
Pre-Training of Equivariant Graph Matching Networks with Conformation Flexibility for Drug Binding.Adv Sci (Weinh). 2022 Nov;9(33):e2203796. doi: 10.1002/advs.202203796. Epub 2022 Oct 6. Adv Sci (Weinh). 2022. PMID: 36202759 Free PMC article.
-
SLC4A11 function: evidence for H+(OH-) and NH3-H+ transport.Am J Physiol Cell Physiol. 2020 Feb 1;318(2):C392-C405. doi: 10.1152/ajpcell.00425.2019. Epub 2019 Nov 27. Am J Physiol Cell Physiol. 2020. PMID: 31774702 Free PMC article.
-
How structural elements evolving from bacterial to human SLC6 transporters enabled new functional properties.BMC Biol. 2018 Mar 14;16(1):31. doi: 10.1186/s12915-018-0495-6. BMC Biol. 2018. PMID: 29540172 Free PMC article.
References
-
- DeFelice LJ. Transporter structure and mechanism. Trends Neurosci. 2004;27(6):352–359. - PubMed
-
- Jardetzky O. Simple allosteric model for membrane pumps. Nature. 1966;211(5052):969–970. - PubMed
-
- Forrest LR, Krämer R, Ziegler C. The structural basis of secondary active transport mechanisms. Biochim Biophys Acta. 2011;1807(2):167–188. - PubMed
Publication types
MeSH terms
Substances
Grants and funding
LinkOut - more resources
Full Text Sources
Other Literature Sources