The nascent polypeptide-associated complex is a key regulator of proteostasis
- PMID: 23604074
- PMCID: PMC3655472
- DOI: 10.1038/emboj.2013.87
The nascent polypeptide-associated complex is a key regulator of proteostasis
Abstract
The adaptation of protein synthesis to environmental and physiological challenges is essential for cell viability. Here, we show that translation is tightly linked to the protein-folding environment of the cell through the functional properties of the ribosome bound chaperone NAC (nascent polypeptide-associated complex). Under non-stress conditions, NAC associates with ribosomes to promote translation and protein folding. When proteostasis is imbalanced, NAC relocalizes from a ribosome-associated state to protein aggregates in its role as a chaperone. This results in a functional depletion of NAC from the ribosome that diminishes translational capacity and the flux of nascent proteins. Depletion of NAC from polysomes and re-localisation to protein aggregates is observed during ageing, in response to heat shock and upon expression of the highly aggregation-prone polyglutamine-expansion proteins and Aβ-peptide. These results demonstrate that NAC has a central role as a proteostasis sensor to provide the cell with a regulatory feedback mechanism in which translational activity is also controlled by the folding state of the cellular proteome and the cellular response to stress.
Conflict of interest statement
The authors declare that they have no conflict of interest.
Figures
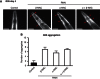
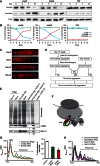
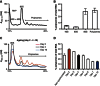
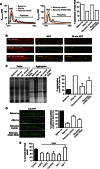
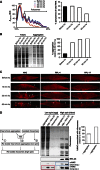
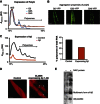
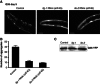
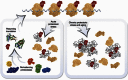
Comment in
-
Ribosome-associated chaperones act as proteostasis sentinels.Cell Cycle. 2013 Aug 1;12(15):2335-6. doi: 10.4161/cc.25703. Epub 2013 Jul 15. Cell Cycle. 2013. PMID: 23856581 Free PMC article. No abstract available.
Similar articles
-
A genetic screening strategy identifies novel regulators of the proteostasis network.PLoS Genet. 2011 Dec;7(12):e1002438. doi: 10.1371/journal.pgen.1002438. Epub 2011 Dec 29. PLoS Genet. 2011. PMID: 22242008 Free PMC article.
-
Conformational flexibility within the nascent polypeptide-associated complex enables its interactions with structurally diverse client proteins.J Biol Chem. 2018 Jun 1;293(22):8554-8568. doi: 10.1074/jbc.RA117.001568. Epub 2018 Apr 12. J Biol Chem. 2018. PMID: 29650757 Free PMC article.
-
Ribosome-associated chaperones as key players in proteostasis.Trends Biochem Sci. 2012 Jul;37(7):274-83. doi: 10.1016/j.tibs.2012.03.002. Epub 2012 Apr 13. Trends Biochem Sci. 2012. PMID: 22503700 Review.
-
The nascent polypeptide-associated complex is essential for autophagic flux.Autophagy. 2014 Oct 1;10(10):1738-48. doi: 10.4161/auto.29638. Epub 2014 Jul 18. Autophagy. 2014. PMID: 25126725 Free PMC article.
-
How Does the Ribosome Fold the Proteome?Annu Rev Biochem. 2020 Jun 20;89:389-415. doi: 10.1146/annurev-biochem-062917-012226. Annu Rev Biochem. 2020. PMID: 32569518 Review.
Cited by
-
The deubiquitinase USP10 protects pancreatic cancer cells from endoplasmic reticulum stress.NPJ Precis Oncol. 2022 Dec 21;6(1):93. doi: 10.1038/s41698-022-00336-x. NPJ Precis Oncol. 2022. PMID: 36543867 Free PMC article.
-
Interrelation between protein synthesis, proteostasis and life span.Curr Genomics. 2014 Feb;15(1):66-75. doi: 10.2174/1389202915666140210210542. Curr Genomics. 2014. PMID: 24653664 Free PMC article.
-
Protein folding in the cell, from atom to organism.FASEB J. 2014 Dec;28(12):5034-8. doi: 10.1096/fj.14-1202ufm. FASEB J. 2014. PMID: 25451678 Free PMC article. No abstract available.
-
Extensive stage-regulation of translation revealed by ribosome profiling of Trypanosoma brucei.BMC Genomics. 2014 Oct 20;15(1):911. doi: 10.1186/1471-2164-15-911. BMC Genomics. 2014. PMID: 25331479 Free PMC article.
-
Analysis of the interplay of protein biogenesis factors at the ribosome exit site reveals new role for NAC.J Cell Biol. 2015 Jul 20;210(2):287-301. doi: 10.1083/jcb.201410086. J Cell Biol. 2015. PMID: 26195668 Free PMC article.
References
-
- Beatrix B, Sakai H, Wiedmann M (2000) The alpha and beta subunit of the nascent polypeptide-associated complex have distinct functions. J Biol Chem 275: 37838–37845 - PubMed
-
- Bloss TA, Witze ES, Rothman JH (2003) Suppression of CED-3-independent apoptosis by mitochondrial betaNAC in Caenorhabditis elegans. Nature 424: 1066–1071 - PubMed
Publication types
MeSH terms
Substances
Grants and funding
LinkOut - more resources
Full Text Sources
Other Literature Sources
Medical